RNA molecule The present invention relates to RNA molecules, and particularly, although not exclusively, to methods for preparing RNA molecules, and to methods for translating RNA molecules into protein. The invention extends to improved methods for forming RNA by in vitro transcription, and to the resultant RNA molecules. Furthermore, the invention relates to novel methods for enhancing the expression and/or translation of RNA, i.e. protein expression, and to methods for improving the stability of an RNA molecule. The invention also involves reducing the activation of innate sensing, interferon generation and/or degradation of an RNA molecule in a host. The invention also incorporates the use of the RNA molecules in vaccines and other therapeutic pharmaceutical compositions, and their use in immunisation and therapy, such as RNAi, gene therapy, gene editing and protein replacement. In particular, the invention relates to locked nucleotides and locked nucleic acid (LNA)-RNA molecules, and the use or incorporation of such locked nucleotides and LNA-RNA molecules in any of the above applications. Locked nucleotides and corresponding locked nucleic acids (LNA) are a modified version of RNA nucleotides where the ribose moiety is modified by a bridge between the 2’ oxygen and 4’ carbon, displacing the hydrogen group from the 2’OH of ribose. First synthesised in the late 1990’s [1,2], this locks the ribose in a 3’-endo (North) conformation, reducing the conformational flexibility of the ribose, and increasing the local organization of the phosphate backbone. As a result, the ribose ring is "locked" in an ideal conformation for Watson-Crick binding. For locked RNA improved hydrophobic interactions between bases increases base staking, an unwinding of the LNA helix relative to RNA, widening of the major groove with an enlarged helical pitch [3-5]. For each incorporated LNA monomer in an oligonucleotide, the melting temperature (Tm) of a duplex increases by 2–8°C, stabilising double stranded LNA- RNA sequences [6, 7]. Such changes in structure likely influence recognition of RNA binding proteins associated with innate RNA recognition. The first LNA compounds, introduced in the late 1990s, are bicyclic nucleotide analogues in which the furanose ring is modified by the introduction of a methylene group linking the 2’-oxygen and the 4’-carbon (2’-O,4’-methylene-d-ribofuranosyl nucleotides) [2,9]. Subsequent derivatives of LNA, include 2’-O,4’-aminoethylene bridged nucleic, 2’-O,4’-C-ethylene-bridged nucleic acid (ENA), 2’-O,4’-C-
methylenoxymethylene-bridged nucleic 2'-N-methanesulfonyl-2'-amino-locked nucleic acid (see Figure 1) [8]. When used to make nucleic acid sequences, these are referred to as “locked nucleic acid” (LNA) or “bridged nucleic acid” (BNA), and sometimes inaccessible RNA [9]. The abbreviation LNA is used interchangeably to indicate locked nucleic acid nucleotides (LNA-NTs), and Locked RNA (LNA-RNA) or Locked DNA (LNA-DNA). Locked NTs and LNAs have been widely used to stabilise short oligonucleotides sequences generated by synthetic synthesis [9]. The inclusion of LNA-NTs is reported to increase oligonucleotide stability, resistance to ribonucleases and reduce innate recognition by cellular RNA binding proteins. The improved stability is thought to be mediated by the locking of the 2’ oxygen, removing its nucleophilic potential to hydrolyse the phosphate linkage between RNA nucleotides [10,11]. The increased resistance to ribonucleases and reduced innate recognition may be mediated by changes in NT/oligonucleotide structure reducing recognition by ribonuclease and innate sensing proteins [3-5, 12, 13]. In many extracellular fluids, RNA degradation is primarily mediated by endoribonucleases that cleave RNAs at certain single-stranded dinucleotide motifs (UA/UA, CA/UG) [14] likely exposed by random thermal fluctuations. LNA modifications of these motifs can effectively enhance RNA nuclease resistance [15-20] while wider LNA modification may enhance nuclease resistance in a sequence independent manner as enhancement of thermostability will make exposure of single stranded motifs to RNase less likely [21]. LNA nucleotides are often used together with other RNA and DNA NTs when synthesised as short oligonucleotides as “miximers” or “gapmers” to fine tune anti-sense function [9]. Although it has not been previously possible to generate LNA-RNA sequences >100 NTs, it can be assumed that LNA-NTs would provide similar properties to longer RNA sequences could they be generated. RNA is composed by the nucleotides ATP, CTP, GTP and UTP, however, a wide range of natural and synthetic modified nucleotides exist [29]. TTP is associated with DNA but has also been used in the generation of locked oligonucleotides. Furthermore, 5meCTP is often used in locked oligonucleotides as it is thought to improve complex stability due to enhanced stacking [30]. Importantly, however, LNAs have never been assessed for their ability to encode and be translated into proteins by cellular ribosomes. This stems from the assumption that the locked conformation and the lack of accessible two prime OH group on the ribose sugar would not represent the required native conformation of RNA to be functional with respect to ribosomal translation. Indeed, locked nucleic acids
are described as an “RNA mimic” or nucleic acids, also known as xeno nucleic acids, XNAs, representing an artificial chemistry that does not exist in nature [21]. RNA vaccine and biotherapeutics have recently come of age, showing successful utility in the COVID-19 pandemic. The two lead COVID-19 candidates manufactured by Moderna and Pfizer both utilise the incorporation of N1-methyl-pseudouridine, a synthetic modified version of the nucleotide triphosphate (NTP) UTP [24]. This is used to reduce triggering of innate pathways that can restrict the expression of encoded proteins rendering the RNA ineffective with respect to therapeutic or vaccine indications [25,26]. The exact mechanism for improved expression is not fully understood and may be related to the suppression of highly immunogenic double stranded RNA (dsRNA) during in vitro transcription [27]. This approach, however, provides little or no advantage in the context of self-amplifying RNA (saRNA). Furthermore, the use of methyl-pseudouridine may not be optimal. Indeed, most cellular receptors detect most double-stranded RNA (dsRNA) structure by contacting the ribose backbone with minimal interaction with the bases. In this respect, ribose base modifications have been predicted to have beneficial effects, e.g. Locked-NTs in relation to innate recognition [12-14]. However, efficient incorporation of LNA-NTs in mRNA or saRNA in sequences over 100nt has not previously been achievable. Furthermore, while previous studies have indicated that use of LNA-NTs can reduce innate recognition of short oligonucleotides, no-one has previously determined whether the locked xeno-nucleic acid can be translated by cellular ribosomes. Indeed, the non- native conformation of LNA would suggest that this would be unlikely and non-obvious given that this has not been assessed in the 30 years since its discovery. Replacement of natural ribose with a ribose moiety modified by a bridge between the 2’ oxygen and 4’ carbon, displacing the hydrogen group from the 2’OH of ribose is known to be less immunogenic and demonstrate greater stability in the context of short synthetically generated short oligonucleotides (<100nt). The locked modification consists of 2’ oxygen and 4’ carbon bridge of the ribose and can therefore occur in all four nucleotides and other non-canonical nucleotides. LNA-RNA has been generated for short RNA sequences, such as aptamers (<25nt in length), utilising in vitro transcription methods that maximise the synthesis of modified RNA from RNA polymerases such as T7 RNA polymerase [22, 23]. However, bottlenecks presently exist
for generating longer LNA-RNA . instance, strict transcription reaction mixture optimisations are required to support high and wide-ranging percentages of nucleotide substitution of wild-type (i.e. non-modified) NTPs. Furthermore, even the generation of LNA-RNA oligonucleotides is currently inefficient, cannot accommodate substitution of all four NTPs and can likely be improved [22,23]. Overcoming these bottlenecks could improve the in vivo applications and potential of LNA-RNAs intended for use as vaccines or biotherapeutics. As discussed above, locked nucleic acid does not exist in nature and is an artificial mimic of natural RNA, with a structure that is highly distorted relative natural RNA [3- 5]. Previously it has not been possible to make LNA-RNA of sufficient size to encode proteins and its non-native nature, in particularly lack of an accessible 2’ ribose OH group and distorted shape, indicate that the ability to engage cellular ribose machinery and generate function proteins would be considered highly unlikely. In this respect LNA-RNA is closer to that of DNA that lacks a 2’ ribose OH group and while able to bind ribosomes is unable to undergo translational elongation required to synthesise proteins [28]. There is, therefore, a need to overcome the problems in the art, and provide improved methods for preparing a modified RNA molecule (for example, mRNA or self- amplifying RNA), which incorporates modified, non-natural locked ribose nucleotides. There is also a need to provide improved methods, when using LNA-NTs, for enhancing RNA stability, expression and translation, as well as reducing the activation of innate sensing, interferon generation and/or RNA degradation. In addition, there is a need to apply the use of such LNA-RNA molecules in improved vaccines and improved therapeutic pharmaceutical compositions, such as RNAi, gene therapy, gene editing and protein replacement. To date, it has not been possible to make RNA sequences by in vitro transcription greater than 25 nucleotides (25nt), using single locked nucleotides [22,23] and not at all with all four RNA locked NTs. Thus, while widely used for short antisense RNA (<100 nucleotides), generated by synthetic processes, no-one has been able to generate sequences of sufficient length to encode functional proteins. Surprisingly, however, the inventors have found that locked nucleotides (such as those shown in Figure 2B) can be effectively incorporated into RNA sequences of greater than 25 nucleotides by in vitro transcription, and that these can be translated by ribosomes within living cells,
generating functional protein. Importantly, the inventors have now observed that the use of individual and combinations of locked nucleotides increases both the level and duration of protein expression in transduced cells. Furthermore, the inventors have demonstrated that different combinations of LNA-NTs can modulate the innate cytokine response to naked unmodified RNA induced when delivered into the cytoplasm of cells. This new approach is likely applicable to all forms of encoding RNA, greater than 100 nucleotides, including messenger RNA, self-amplifying RNA saRNA), circular RNA, or to any other form of RNA of a length greater than 100 nucleotides. The inventors have surprisingly shown that the generation of RNA containing locked nucleotides by in vitro transcription is promoted by high levels of magnesium ions, ideally as magnesium acetate greater than 20 mM, optimally greater than 40 mM (see Figure 2a) or magnesium chloride greater than 20 mM, optimally 40 mM (see Figure 2b). This opens the possibility for enzymatic generation of any RNA sequence of any size using locked nucleotides. This greatly improves the potential economics of making locked RNA sequence of any size, given that enzymatic production of RNA is considered considerably cheaper, and more efficient than current synthetic solid state methods for generating short locked RNA sequences used for multiple applications including antisense RNA, RNA aptamers, external guide sequences, ribozymes, short interfering RNA [2,9,16,17], and, as well as longer RNA greater than 25 nucleotides, such as long non-coding RNA (Lnc-RNA), or encoding RNA: including mRNA; self-amplifying RNA; circular RNA; or any other form of longer RNA. Thus, in a first aspect of the invention, there is provided one or more locked nucleotide, for use in: (i) enhancing the expression and/or translation of an RNA molecule comprising the one or more locked nucleotide; (ii) enhancing the stability of an RNA molecule comprising the one or more locked nucleotide; and/or (iii) reducing the activation of innate sensing, interferon generation and/or degradation of an RNA molecule comprising the one or more locked nucleotide. In a second aspect, there is provided a method of:
(i) enhancing the expression of an RNA molecule comprising one or more locked nucleotide; (ii) enhancing the stability of an RNA molecule comprising one or more locked nucleotide; and/or (iii) reducing the activation of innate sensing, interferon generation and/or degradation of an RNA molecule comprising one or more locked nucleotide, wherein the method comprises incorporating one or more locked nucleotide into the RNA molecule, to thereby enhancing the expression and/or translation of the RNA molecule comprising the one or more locked nucleotide, enhancing the stability of the RNA molecule comprising the one or more locked nucleotide, and/or reducing the activation of innate sensing, interferon generation and/or degradation of the RNA molecule comprising the one or more locked nucleotide. Enhancing expression of translation of a modified RNA molecule is an important aspect of the invention. Accordingly, in a third aspect, there is provided a method of enhancing the translation of an RNA molecule, the method comprising translating an RNA molecule comprising one or more locked nucleotide, wherein the level of translation in the presence of the one or more locked nucleotide is greater than the level of translation in the absence of the one or more locked nucleotide. In a fourth aspect, there is provided the use of one or more locked nucleotide in an RNA molecule, for enhancing the translation of the RNA molecule. In a fifth aspect, there is provided one or more locked nucleotide in an RNA molecule, for use in enhancing the translation of the RNA molecule. Any of the methods or uses described herein may be carried out in vivo, in vitro or ex vivo. However, most preferably the methods are carried out in vivo. As described in the Examples, the inventors have demonstrated that incorporation of locked nucleotides (e.g. as shown in Figure 2B) to form LNA as LNA-RNA (either LNA- mRNA or LNA-saRNA) can be achieved by in vitro transcription, promoted by high levels of magnesium ions, ideally magnesium acetate greater than 20 mM, optimally greater than 40 mM (see Figure 2a) or magnesium chloride greater than 20 mM,
optimally at 40mM (see Figure 2b). The were surprised to observe that LNA- RNA can be translated, enhance the expression of the RNA, both self-amplifying RNA (saRNA) and mRNA, and generate functional protein in interferon-responsive cells relative to unmodified RNA, which does not comprise LNAs. Substitution of up to 90% of individual nucleotides (ATP, CTP, GTP, UTP, TTP or 5meCTP) with locked nucleotides surprisingly enhances mRNA expression of encoded firefly luciferase (fLuc) (which acts as a marker or surrogate of protein expression) by up to 4-fold in HEK293T cells that have impaired innate immunity sensing (see Figures 3 and 4). These data suggest that individual locked nucleotides (LNA-NTPs) have the surprising potential to enhance expression in the absence of innate recognition. Substitution of individual nucleotides (NTPs) with locked nucleotides (NTPs) enhances fLuc expression in HeLa epithelial cells by up to 10-fold at 24 hours (LNA-UTP) and 60-fold (LNA-UTP) at 48h. These data indicate that the incorporation of LNAs enhance both the magnitude and duration of expression in epithelial cells with intact innate sensing pathways (see Figure 5 and 6), where the impact hierarchy on expression for the individual NTPs is UTP=TTP>CTP=5meCTP>ATP>GTP. Furthermore, substitution of individual nucleotides (NTPs) with locked nucleotides (NTPs) enhances fLuc expression in THP-1 monocytic cells by up to 10-fold at 24 hours (LNA-UTP) and 20-fold (LNA-UTP) at 48h (Figure 7&8). These data confirm that the incorporation of LNAs enhance both the magnitude and duration of expression in cells with intact innate sensing pathways, in this case demonstrated using a monocytic cell line (see Figures 7 and 8). These cells demonstrated the same hierarchy of impact on expression for the individual NTPs where UTP=TTP>CTP=5meCTP>ATP>GTP. It was also surprisingly observed that substitution of individual nucleotides (NTPs) with LNAs was able to influence the secretion profile of a panel of cytokines in supernatants harvested from transfected THP-1 cells (see Figure 9). In particular, LNA- GTP was able to reduce IFN-alpha, while LNA-GTP and LNA-UTP reduced IP-10, and all four LNA-NTPs (ATP, CTP, GTP, UTP) reduced MCP1 and MCP2 with a hierarchy of GTP>UTP>CTP>ATP. These data demonstrate that individual LNA-NTPs impact on innate recognition and associated cytokine induction. Moreover, experiments in human skin explants and in vivo murine studies have shown that LNA modified RNA increased both the magnitude and duration of expression in
ex-vivo human skin explants and also in where expression of LNA modified saRNA was greater than mRNA. Additionally, these data indicate that LNA modification reduces the expression of cytokines and chemokines induced by mRNA and saRNA injection in vivo. For example, such cytokines and chemokines can include CRO-alpha, IFN-alpha 2a, IFN-beta, IFN-gamma, IP-10, MCP-1, MCP-2, MCP-3, MIP-1 alpha, and/or MIP-1 beta. In summary, these data are highly unexpected given that LNA-RNA has never been considered or assessed for translation and protein production, being a non-native RNA mimic (or xeno-nucleic acid) that is more analogous to DNA, also thought to be ineffective with respect to protein translation [28] where elongation is only evident in the presence of neomycin [31]. Hence, in a sixth aspect of the invention, there is provided a method of preparing a locked nucleic acid RNA (LNA-RNA) molecule, wherein the method comprises contacting, in the presence of at least 20mM magnesium ions, (i) a template nucleic acid sequence, (ii) an RNA polymerase, and (iii) a plurality of nucleotide triphosphates (NTPs), one or more of which comprises a locked nucleotide, wherein the RNA polymerase transcribes the template nucleic acid sequence to form a LNA-RNA molecule comprising at least 25 nucleotides. In a seventh aspect, there is provided use of 20mM magnesium ions in a transcription reaction to prepare a locked nucleic acid RNA (LNA-RNA) molecule comprising at least 25 nucleotides. Advantageously, the inventors have devised novel and innovative in vitro transcription reaction conditions for use in the methods of the invention. These reaction conditions include higher than previously used magnesium ion concentrations (i.e. >20mM, preferably >40mM), and therefore enable significant levels of replacement of wild-type (i.e. unmodified) nucleotides in the resultant LNA-RNA molecule with locked nucleotides or LNAs. To date, it has not been possible to generate mRNA or saRNA sequences by in vitro transcription, of sizable length (>25nt, >50nt, >100nt, >500nt, >1000nt, or more) where single or multiple wild-type unmodified nucleotides are replaced by locked nucleotides or LNAs, and where the RNA molecule is expressed and translated into a functional protein. It will be appreciated that the smallest human protein is reported to be 44 amino acids long (i.e.132 nucleotides long excluding UTRs
and a poly A tail), and that the smallest protein is only 51 amino acids long (i.e. insulin). Accordingly, the invention described herein means that it is now possible to produce functional proteins comprising locked nucleotides, ither by mRNA or saRNA. The in vitro transcription reaction mixture in the methods of the invention requires the use of >20mM magnesium ions and is demonstrably higher than some conventional in vitro transcription methods. LNA-RNAs, both mRNA and saRNA, synthesised from the methods described herein exhibit significantly enhanced expression in interferon responsive cells. This is highly likely to enhance the in vivo potential of RNA vaccines (for example, expressing microbial antigens for immunizing against infectious micro- organisms, such as viruses and bacteria etc., and also cancer vaccines expressing oncogenic antigens) as well as RNA biotherapeutics (for example, RNAi, protein replacement, gene editing and gene therapy etc.) whether delivered as mRNA or saRNA. Preferably, the method comprises the use of at least 25mM magnesium ions, preferably at least 30mM magnesium ions, more preferably at least 35mM magnesium ions, preferably at least 40mM magnesium ions, and even more preferably at least 5omM magnesium ions. Preferably, the method comprises the use of at least 60mM magnesium ions, more preferably at least 70mM magnesium ions, and even more preferably at least 75mM magnesium ions. Preferably, the method comprises the use of at least 80mM magnesium ions, more preferably at least 85mM magnesium ions, more preferably at least 90mM magnesium ions, and even more preferably at least 100mM magnesium ions. Preferably, the method comprises the use of less than 120mM magnesium ions, preferably less than 100mM magnesium ions, more preferably less than 90mM magnesium ions, preferably less than 80mM magnesium ions, and even more preferably less than 75mM magnesium ions. Preferably, the method comprises the use of between 50mM and 100mM magnesium ions, more preferably between 60mM and 95mM magnesium ions, more preferably between 65mM and 90mM magnesium ions, and even more preferably between 70mM and 80mM magnesium ions. Preferably, the method comprises the use of between 71mM and 79mM magnesium ions, more preferably between 73mM and 77mM magnesium ions.
The magnesium ions may be provided method as Mg
2+ ions. The magnesium ions may be provided as magnesium acetate, magnesium chloride, magnesium citrate, magnesium sulphate, magnesium gluconate, or magnesium lactate, and so on. As described in the Examples, the inventors observed increased RNA expression when the magnesium ions were provided in the form of magnesium acetate, and to a lesser extent, magnesium chloride, and this was totally unexpected. Hence, preferably the magnesium ions are provided as magnesium acetate. Preferably, the magnesium ions are not provided as magnesium chloride. As such, in an embodiment in which the method uses magnesium acetate, the method comprises the use of between 30mM and 90mM magnesium acetate, more preferably between 40mM and 80mM magnesium acetate, more preferably between 50mM and 70mM magnesium acetate, and even more preferably between 60mM and 75mM magnesium ions. However, in an embodiment in which the method uses magnesium chloride, the method comprises the use of between 25mM and 55mM magnesium chloride, more preferably between 30mM and 50mM magnesium chloride, more preferably between 35mM and 45mM magnesium chloride, and even more preferably between 38mM and 42mM magnesium chloride. The RNA molecule may be single-stranded or double-stranded. The skilled person would appreciate that when the nucleic acid is double-stranded, for example double-stranded RNA, “bases in length” will refer to the length of base pairs. The RNA molecule may be coding. For example, coding RNA may be used for therapeutic applications and vaccine applications. The RNA molecule may be non-coding. For example, non-coding RNA may be used for RNAi applications. The RNA molecule may be selected from a group consisting of: messenger RNA (mRNA), micro RNA (miRNA); short interfering RNA (siRNA); short hairpin RNA (shRNA); anti- sense RNA; RNA aptamers; self-amplifying RNA (saRNA); interference RNA (RNAi); non-coding RNA; circular RNA; and small RNA.
Preferably, the RNA molecule is self- RNA (saRNA) or messenger RNA (mRNA). In an embodiment, the RNA molecule may not comprise a cap. However, preferably, the RNA molecule comprises a cap. Preferably, the one or more locked nucleotide (or LNA) is not part of the cap. In other words, preferably the cap does not comprise the one or more locked nucleotide. The RNA molecule may be self-amplifying RNA (saRNA). The skilled person would appreciate that self-amplifying RNAs may contain the basic elements of mRNA (a cap, 5’ UTR, 3’UTR, an IRES, a viral polymerase, and poly(A) tail of variable length), but may be considerably longer (for example 9-12 kb). Examples 12-14 describe saRNA comprising locked nucleotides or LNA-NTPs, i.e. LNA-saRNA. The RNA molecule may be messenger RNA (mRNA). mRNA may contain the basic elements of a cap, 5’ UTR, 3’UTR, IRES, and poly(A) tail of variable length. Examples 1-11 describe mRNA comprising locked nucleotides or LNA-NTPs, i.e. LNA-mRNA. The RNA molecule (which may be RNAi, saRNA or mRNA) may be at least 20, 21, 22 or 23 bases in length. The RNA molecule may be at least 24, 25, 26 or 27 bases in length. The RNA molecule may be at least 28, 29, 30, or 31 bases in length. The RNA molecule may be at least 32, 33, 34 or 35 bases in length. The RNA molecule may be at least 36, 37, 38 or 39 bases in length. The RNA molecule may be at least, at least 40, 41, 42, 43, 44 or 45 bases in length. The RNA molecule may be at least 46, 47, 48 or 49 bases in length. The RNA molecule (which may be RNAi, saRNA or mRNA) may be at least at least 50 bases in length, at least 60 bases in length, at least 75 bases in length, at least 100 bases in length, at least 200 bases in length, at least 300 bases in length, at least 400 bases in length, at least 500 bases in length, at least 600 bases in length, at least 700 bases in length, at least 800 bases in length, or at least 900 bases in length. The RNA molecule, most preferably saRNA or mRNA, may be at least 1000 bases in length, at least 2000 bases in length, at least 3000 bases in length, at least 4000 bases in length, at least 5000 bases in length, at least 6000 bases in length, at least 7000 bases in length, at least 8000 bases in length, at least 9000 bases in length, at least 10,000 bases in length, at least 11,000 bases in length or at least 12000 bases in length.
In one embodiment, RNA molecule is at least 6000 bases in length. In one embodiment, the RNA, most preferably saRNA or mRNA, is at least 6000 bases in length. In a preferred embodiment, the saRNA is at least 6000 bases in length. The RNA molecule, most preferably saRNA or mRNA, may be between 5000 and 20,000 bases in length, between 6000 and 15,000 bases in length, between 7000 and 14,000 bases in length, between 7500 and 13,000 bases in length, between 8000 and 12,000 bases in length, between 8500 and 11,000 bases in length, between 9000 and 10,000 bases in length. The methods of the invention may be carried out in vivo, in vitro or ex vivo. However, most preferably the methods are carried out in vitro. Preferably, the methods comprise in vitro transcription (IVT). Preferably, the method comprises the use of an RNA polymerase, which may be selected from a group consisting of: T7; T3; SP6; KP34; Syn5; or other DNA-dependent RNA polymerase; or a mutated variant of any of these RNA polymerases. Each of these RNA polymerases is able to maximise the synthesis of the modified RNA. Preferably, however, the method comprises the use of T7 RNA polymerase or a variant thereof. T7 RNA polymerase variants may include the following mutations (singly or in combination) associated with increased processivity and/or tolerance for incorporation of modified bases, specifically R425C, K631R, S633P, Y639F, Y639V, S641A, H784A, H784S, and/or H784G. Preferably, the plurality of nucleotide triphosphates (NTPs) are selected from the group consisting of ATP, GTP, CTP, TTP and/or UTP. NTPs are the building blocks of RNA. Preferably, the method comprises the use of substantially equal proportions of each of ATP, GTP, CTP, TTP and/or UTP. However, in some embodiments, the method may comprise different ratios between each of ATP, GTP, CTP, TTP and/or UTP, examples of which are given below. This will depend on the sequence of the template nucleic acid and resultant RNA molecule to be transcribed therefrom. Preferably, the methods comprise the use of the plurality of nucleotide triphosphates at a concentration of at least 1mM, 2mM, 3mM or 4mM. Preferably, the methods comprise the use of the plurality of nucleotide triphosphates at a concentration of at
least 5mM, 6mM or 7mM. More methods comprise the use of the plurality of nucleotide triphosphates at a concentration of at least 8mM, 9mM, 10mM. Suitably, the methods comprise the use of the plurality of nucleotide triphosphates at a concentration of greater than 10mM. For example, the methods may comprise the use of the plurality of nucleotide triphosphates at a concentration of at least 12mM, 14mM, or 16mM. The methods may also comprise the use of the plurality of nucleotide triphosphates at a concentration of at least 18mM, 20mM, or 22mM. In other embodiments, the methods may comprise the use of the plurality of nucleotide triphosphates at a concentration of at least 25mM, 50mM, 75mM or 100mM. It will be appreciated that the one or more locked nucleotide may comprise a ribose moiety modified by a bridge between the 2 and 4 positions or the 2’ and 4’ positions in the ribose sugar. Thus, the one or more locked nucleotide preferably comprises a bridge between the 2’ and 4’ positions in the ribose sugar. The bridge may displace the hydrogen groups from the 2’OH of the ribose and from the 4’ position of the ribose. The one or more locked nucleotide may be represented herein by formula [I]: wherein Base is a nucleobase;

L is a C1-5 alkylene; X
1 is O, CH2, CO, NR
5, Se, S, SO or SO2; X
2 is absent, or is CO, O, NR
5, Se, S, SO or SO2; one of R
1 and R
2 is a phosphate linker, a modified phosphate linker or a 5 to 10 membered heteroarylene, wherein the phosphate linker, the modified phosphate linker or the 5 to 10 membered heteroarylene is a linker between the rest of the locked nucleotide of formula [I] and an attachment point to a first adjacent nucleotide in the RNA molecule, and the other of R
1 and R
2 is a bond to a second adjacent nucleotide in the RNA molecule; R
3 and R
4 are independently H or C
1-6 alkyl; and .
Preferably, the one or more locked nucleotide may be represented herein as formula [Ia]:
The nucleobase may be a primary or a A primary nucleobase may be a purine or a pyrimidine. A purine may be adenine, guanine or hypoxanthine. A pyrimidine may be cytosine, uracil or thymine. The term “modified nucleobase” may be understood by the skilled person. For instance, the modified nucleobase may have the structure of a primary nucleobase with one or more modifications. The one or more modifications may include one or more of: - replacing a hydrogen and/or an NH2 group in the primary nucleobase with an optionally substituted C1-6 alkyl, an optionally substituted C2-6 alkenyl, an optionally substituted C
2-6 alkynyl, OR
15, NR
15R
16, COOR
15, CONR
15R
16 or a halogen; - replacing a double bond with a single bond; - replacing a CH in the structure with an N; - replacing an N in the structure with a CR
15; - replacing an O in the structure with an NR
15, S, Se or CR
15R
16; and/or - the nucleobase is attached to the rest of the locked nucleotide of formula [I] at a different point to the point where the primary nucleobase would typically be attached; wherein R
15 and R
16 are independently H, an optionally substituted C1-6 alkyl, an optionally substituted C1-6 alkenyl, an optionally substituted C1-6 alkynyl or an optionally substituted C6-12 aryl, or R
15 and R
16 together with the atom to which they are bonded for an optionally substituted 3 to 10 membered cycloalkyl or an optionally substituted 3 to 10 membered heterocyclyl.
An optionally substituted alkyl, alkenyl may be unsubstituted or substituted with one or more of halogen, OR
17, COOR
17, CONR
17R
18 and NR
17R
18, wherein R
17 and R
18 are H, C
1-6 alkyl, C
2-6 alkenyl or C
2-6 alkynyl. An optionally substituted aryl, cycloalkyl or heterocycyl may be unsubstituted or substituted with one or more of halogen, OR
17, COOR
17, CONR
17R
18 and NR
17R
18, wherein R
17 and R
18 are H, C1-6 alkyl, C2-6 alkenyl or C2-6 alkynyl. A halogen may be fluorine, chlorine or bromine. A locked nucleic acid (LNA) with a modified uracil nucleobase may include pseudouridine (ψ), pyridin-4-one ribonucleoside, 5-aza-uridine, 6-aza-uridine, 2-thio- 5-aza-uridine, 2-thio-uridine (s
2U), 4-thio-uine (s4U), 4-thio-pseudouridine, 2-thio- pseudouridine, 5-hydroxy-uridine (ho5U), 5-aminoallyl- uridine, 5-halo-uridine (e.g., 5-iodo~uridine or 5-bromo-uridine), 3-methyl-uridine (m
3U), 5- methoxy-uridine (mo
5U), uridine 5-oxyacetic acid (cmo
5U), uridine 5-oxyacetic acid methyl ester (mcmo
5U), 5-carboxymethyl-uridine (cm
5U), 1-carboxymethyl-pseudouridine, 5- carboxyhydroxymethyl-uridine (chm
5U), 5-carboxyhydroxymethyl-uridine methyl ester (mchm
5U), 5-methoxycarbonylmethyl-uridine (mcm
5U), 5- methoxycarbonylmethyl-2-thio-uridine (mcm
5sU), 5-aminomethyl-2-thio-uridine (nmVU), 5-methylaminomethyl-uridine (mnm
5U), 5- methylaminomethyl-2-thio- uridine (mnm
5s
2U), 5-methylaminomethyl-2-seleno-uridine (mnm
5se
2U), 5- carbamoylmethyl-uridine (ncm
5U), 5-carboxymethylaminomethyl-uridine (cmnm
5U), 5- carboxymethylaminomethyl-2-thio-uridine (cmnm
5s
2U), 5-propynyl-uridine, 1- propynyl- pseudouridine, 5-taurinomethyl-uridine (tm
5U), 1-taurinomethyl- pseudouridine, 5-taurinomethyl-2- thio-uridine^mVU), l-taurinomethyl-4-thio- pseudouridine, 5-methyl-uridine (m
5U, i.e., having the nucleobase deoxythymine), 1- methyl-pseudouridine (m^), 5-methyl-2-thio-uridine (m
5s
2U), 1- methyl-4-thio- pseudouridine (m
1s
4y) 4-thio-1-methyl-pseudouridine, 3-methyl-pseudouridine (m
3y) , 2-thio- 1-methyl-pseudouridine, 1-methyl- 1-deaza-pseudouridine, 2-thio-l -methyl- 1- deaza- pseudouridine, dihydrouridine (D), dihydropseudouridine, 5,6-dihydrouridine, 5-methyl- dihydrouridine (m
5D), 2-thio-dihydrouridine, 2-thio-dihydropseudouridine, 2-methoxy-uridine, 2- methoxy-4-thio-uridine, 4-methoxy-pseudouridine, 4-methoxy- 2-thio-pseudouridine, N1-methyl- pseudouridine, 3-(3-amino-3-carboxypropyl)uridine
(acp
3U), l-methyl-3-(3-amino-3- pseudouridine (acp3y), 5- (isopentenylaminomethyl)uridine (inm
5U), 5- (isopentenylaminomethyl)-2-thio- uridine (inm
5s
2U), a-thio-uridine, 1-thio- uridine, deoxythymidine, 5-(2- carbomethoxyvinyl) uridine, and 5-[3-(l-E-propenylamino)uridine. In some embodiments, LNA-CTP contains a modified nucleobase. Exemplary base modified LNA-CTPs include 5-aza-cytidine, 6-aza-cytidine, pseudoisocytidine, 3- methyl-cytidine (m
3C), N4-acetyl-cytidine (ac
4C), 5-formyl-cytidine (f
5C), N4- methyl- cytidine (m
4C), 5-methyl-cytidine (m
5C), 5-halo-cytidine (e.g., 5-iodo-cytidine), 5- hydroxymethyl-cytidine (hm5C), 1-methyl-pseudoisocytidine, pyrrolo-cytidine, pyrrolo- pseudoisocytidine, 2-thio-cytidine (s
2C), 2-thio-5-methyl-cytidine, 4-thio- pseudoisocytidine, 4-thio-1-methyl-pseudoisocytidine, 4-thio-1-methyl-1-deaza- pseudoisocytidine, 1-methyl-1-deaza- pseudoisocytidine, zebularine, 5-aza-zebularine, 5-methyl-zebularine, 5-aza-2-thio-zebularine, 2- thio-zebularine, 2-methoxy-cytidine, 2-methoxy-5-methyl-cytidine, 4-methoxy-pseudoisocytidine, 4- methoxy- 1-methyl- pseudoisocytidine, lysidine (k
2C), a-thio-cytidine, 1-thio-cytidine. In some embodiments, LNA-ATP contains a modified nucleobase. Exemplary base modified LNA-ATPs include 2-amino-purine, 2, 6-diaminopurine, 2-amino-6-halo- purine (e.g., 2-amino-6-chloro-purine), 6-halo-purine (e.g., 6-chloro-purine), 2- amino-6-methyl-purine, 8-azido-adenosine, 7-deaza-adenine, 7-deaza-8-aza-adenine, 7-deaza-2- amino-purine, 7-deaza-8-aza-2-amino-purine, 7-deaza-2,6-diaminopurine, 7-deaza-8-aza-2,6- diaminopurine, 1-methyl-adenosine (m
1A), 2-methyl-adenine (m
2A), N6-methyl-adenosine(m
6A), 2- methylthio-N6-methyl-adenosine (ms
2m
6A), N6-isopentenyl-adenosine (i
6A), 2-methylthio-N6- isopentenyl-adenosine (ms
2i
6A), N6-(cis-hydroxyisopentenyl)adenosine (io
6A), 2-methylthio-N6- (cis- hydroxyisopentenyl)adenosine (ms
2io
6A), N6-glycinylcarbamoyl-adenosine (g
6A), N6- threonylcarbamoyl-adenosine (t
6A), N6-methyl-N6-threonylcarbamoyl-adenosine (m
6t
6A), 2- methylthio-N6-threonylcarbamoyl-adenosine (ms
2g
6A), N6,N6-dimethyl- adenosine (m
6 2A), N6- hydroxynorvalylcarbamoyl-adenosine (hn
6A), 2-methylthio-N6- hydroxynorvalylcarbamoyl- adenosine (ms
2hn
6A), N6-acetyl-adenosine (ac
6A), 7- methyl-adenine, 2-methylthio-adenine, 2- methoxy-adenine, a-thio-adenosine, 2- amino-N6-methyl-purine, 1-thio-adenosine, 8-azido- adenosine, and N6-(19-amino- pentaoxanonadecyl)-adenosine.
A locked nucleic acid (LNA) with a guanine nucleobase may include inosine (I), 1-methyl-inosine (m
1!), wyosine (imG), methylwyosine (mimG), 4-demethyl- wyosine (imG-14), isowyosine (imG2), wybutosine (yW), peroxywybutosine (o
2yW), hydroxywybutosine (OHyW), undermodified hydroxybutosine (OHyW*), 7-deaza- guanosine, queuosine (Q), epoxyqueuosine (oQ), galactosyl- queuosine (galQ), mannosyl-queuosine (manQ), 7-cyano-7-deaza-guanosine (preQ0), 7- aminomethyl-7- deaza-guanosine (preQi), archaeosine (G
+), 7-deaza-8-aza-guanosine, 6-thio- guanosine, 6-thio-7-deaza-guanosine, 6-thio-7-deaza-8-aza-guanosine, 7-methyl- guanosine (m
7G), 6-thio-7-methyl-guanosine, 7-methyl-inosine, 6-methoxy-guanosine, 1-methyl-guanosine (m
1G) , N2-methyl-guanosine (m
2G), N2,N2-dimethyl-guanosine (m
22G), N2,7-dimethyl-guanosine (m
2'7G), N2, N2,7-dimethyl-guanosine (m
2'2'7G), 8- oxo-guanosine, 7-methyl-8-oxo-guanosine, l-methyl-6- thio-guanosine, N2-methyl-6- thio-guanosine, N2,N2-dimethyl-6-thio-guanosine, a-thio-guanosine, 1-thio-guanosine, 06-methyl-guanosine. The nucleobase may be a naturally-occurring or synthetic derivative of a nucleobase, including pyrazolo[3,4-d]pyrimidines, 5-methylcytosine (5-me-C), 5-hydroxymethyl cytosine, xanthine, hypoxanthine, 2-aminoadenine, 6-methyl and other alkyl derivatives of adenine and guanine, 2-propyl and other alkyl derivatives of adenine and guanine, 2-thiouracil, 2-thiothymine and 2-thiocytosine, 5-propynyl uracil and cytosine, 6-azo uracil, cytosine and thymine, 5-uracil (pseudouracil), 4-thiouracil, 8- halo (e.g., 8-bromo), 8-amino, 8-thiol, 8-thioalkyl, 8-hydroxyl and other 8-substituted adenines and guanines, 5-halo particularly 5-bromo, 5-trifluoromethyl and other 5- substituted uracils and cytosines, 7-methylguanine and 7-methyladenine, 8-azaguanine and 8- azaadenine, deazaguanine, 7-deazaguanine, 3-deazaguanine, deazaadenine, 7- deazaadenine, 3- deazaadenine, pyrazolo[3,4-d]pyrimidine, imidazo[l,5-a]l,3,5 triazinones, 9-deazapurines, imidazo[4,5-d]pyrazines, thiazolo[4,5-d]pyrimidines, pyrazin-2-ones, 1,2,4-triazine, pyridazine; and 1,3,5 triazine. In some embodiments, the modified nucleobase is 5-methyl-cytosine. L may be a straight or branched C
1-5 alkylene. Preferably, L is a straight or branched C
1- 3 alkylene. More preferably, L is -CH2-*, -CH(CH3)-*, -CH2CH2-* or -CH(CH3)CH2-*, where an asterisk indicates a point of attachment to X
2 in embodiments where it is present and to X
1 in embodiments where X
2 is absent. Most preferably, L is CH2.
X
1 may be O, CH2, NR
5, Se or S. In X
1 is O. X
2 may be absent. Alternatively, X
2 may be SO
2. A phosphate linker may be understood to have the following structure: . A modified phosphate linker may have the same structure as a phosphate linker except one or more of the oxygens may have been replaced with a different substitutent. For instance, one or more of the oxygens in the phosphate linker may have been replaced with an NR
13, S, Se, BR
13, BH3, H, an optionally substituted C1-6 alkyl, an optionally substituted C1-6 alkenyl, an optionally substituted C1-6 alkynyl and/or an optionally substituted C6-12 aryl and/or the O- in the phosphate linker may have been replaced with NR
13R
14, CR
13R
14, S-, Se-, BH3, H, an optionally substituted C1-6 alkyl, an optionally substituted C1-6 alkenyl, an optionally substituted C1-6 alkynyl or an optionally substituted C
6-12 aryl wherein R
13 and R
14 are independently H, an optionally substituted C1-6 alkyl, an optionally substituted C1-6 alkenyl, an optionally substituted C1-6 alkynyl or an optionally substituted C6-12 aryl, or R
13 and R
14 together with the atom to which they are bonded for an optionally substituted 3 to 10 membered cycloalkyl or an optionally substituted 3 to 10 membered heterocyclyl. Examples of modified phosphate groups include, but are not limited to, phosphorothioate, phosphoroselenates, boranophosphates, boranophosphate esters, hydrogen phosphonates, phosphoramidates, phosphorodiamidates, alkyl or aryl phosphonates, and phosphotriesters. Phosphorodithioates have both non-linking oxygens replaced by sulfur. The phosphate linker can also be modified by the replacement of a linking oxygen with nitrogen (bridged phosphoramidates), sulfur (bridged phosphorothioates), and carbon (bridged methylene- phosphonates). The a-thio substituted phosphate moiety is provided to confer stability through the unnatural phosphorothioate backbone linkages. Phosphorothioate DNA and RNA have increased nuclease resistance and subsequently a longer half-life in a cellular environment. Phosphorothioate linked modified nucleic acids or mmRNA molecules are expected to also reduce the innate immune response through weaker binding/activation of cellular innate immune molecules.
In specific embodiments, a modified nucleoside includes an alpha-thio-nucleoside (e.g., 5'- 0-(l-thiophosphate)-adenosine, 5'-0-(l-thiophosphate)-cytidine (a-thio-cytidine), 5'- 0-(l-thiophosphate)-guanosine, 5'-0-(l-thiophosphate)-uridine, or 5'-0-(l- thiophosphate)- pseudouridine). Accordingly, one of R
1 and R
2 may ,
, wherein
of formula [I] and indicates the attachment point of the R
1 or R
2 group to a first adjacent nucleotide in the RNA molecule. In embodiments where one of R
1 or R
2 is a 5 to 10 membered heteroarylene, the heteroarylene is preferably a 5 or 6 membered heteroarylene, and more preferably is a 5 membered heteroarylene. The heteroarylene , wherein indicates the attachment point of the R
1 or R
2 group to the
of formula [I] and indicates the attachment point of the R
1 or R
2 group to a first adjacent
in the RNA molecule. Preferably, one of R
1 or R
2 and R
2 is a bond.
R
3 and R
4 may independently be H or C1-3 alkyl, and more preferably are independently H or methyl. In some embodiments, R
3 and R
4 are both H.
In one a bond, R
3 is H, R
4 is H, X
1 is O, X
2 is absent and L is
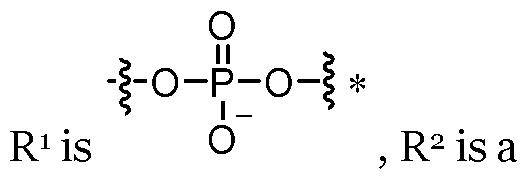
cytosine, 5-methyl-cytosine, guanine, uracil or thymine. Accordingly, preferably the use or method comprises using one or more locked nucleotide selected from a group consisting of: locked adenine; locked cytosine; 5- methyl-cytosine; locked guanine; locked uracil and/or locked thymine. Preferably, the use or method comprises using at least two locked nucleotides selected from a group consisting of: a locked adenine; a locked cytosine; a 5-methyl-cytosine; a locked guanine; a locked uracil and/or a locked thymine. Preferably, the use or method comprises using at least three locked nucleotides selected from a group consisting of: a locked adenine; a locked cytosine; a 5-methyl-cytosine; a locked guanine; a locked uracil and/or a locked thymine. Preferably, the use or method comprises using at least four locked nucleotides selected from a group consisting of: a locked adenine; a locked cytosine; a 5-methyl-cytosine; a locked guanine; a locked uracil and/or a locked thymine. Preferably, the use or method comprises using at least five locked nucleotides selected from a group consisting of: a locked adenine; a locked cytosine; a 5-methyl- cytosine; a locked guanine; a locked uracil and/or a locked thymine. Preferably, the use or method comprises using at least six locked nucleotides selected from a group consisting of: a locked adenine; a locked cytosine; a 5-methyl-cytosine; a locked guanine; a locked uracil and/or a locked thymine. Preferably, one or more of the locked nucleotides used in the invention (e.g. in the in vitro transcription reaction or in the resultant LNA-RNA molecule) are locked, in that they are not naturally occurring. In an embodiment, at least 1%, 2%, 3%, 4% or 5% of the constituent nucleotides in the transcription reaction or in the resultant LNA-RNA molecule may comprise locked nucleotides, wherein the locked nucleotides may comprise locked adenine, locked cytosine, locked guanine, locked thymine and/or locked uracil. However, at least 6%, 7%, 8%, 9% or 10% of the constituent nucleotides in the transcription reaction or in the resultant LNA-RNA molecule may comprise locked nucleotides, wherein the locked nucleotides may comprise locked adenine, locked cytosine, locked guanine, locked
thymine and/or locked uracil. 15%, 17%, 19%, 20% or 25% of the constituent nucleotides in the transcription reaction or in the resultant LNA-RNA molecule may comprise locked nucleotides, wherein the locked nucleotides may comprise locked adenine, locked cytosine, locked guanine, locked thymine and/or locked uracil. Preferably, at least 30%, 35% or 40% of the constituent nucleotides in the transcription reaction or in the resultant RNA molecule are locked, wherein the locked nucleotides may comprise locked adenine, locked cytosine, locked guanine, locked thymine and/or locked uracil. More preferably, at least 45%, 50% or 55% of the constituent nucleotides in the transcription reaction or in the resultant RNA molecule are locked, wherein the locked nucleotides may comprise locked adenine, locked cytosine, locked guanine, locked thymine and/or locked uracil. Even more preferably, at least 60%, 65% or 70% of the constituent nucleotides in the transcription reaction or in the RNA molecule are locked, wherein the locked nucleotides may comprise locked adenine, locked cytosine, locked guanine, locked thymine and/or locked uracil. Still more preferably, at least 75%, 80% or 85% of the constituent nucleotides in the transcription reaction or in the RNA molecule are locked, wherein the locked nucleotides may comprise locked adenine, locked cytosine, locked guanine, locked thymine and/or locked uracil. Most preferably, at least 90%, 95%, 96%, 97%, 98%, 99% or 100% of the constituent nucleotides in the transcription reaction or in the RNA molecule are locked, wherein the locked nucleotides may comprise locked adenine, locked cytosine, locked guanine, locked thymine and/or locked uracil. Preferably, the transcription reaction is an in vitro transcription reaction. In an embodiment, at least 0.01%, 0.02%, 0.03%, 0.04% or 0.05% of the constituent nucleotides in the resultant LNA-RNA molecule may comprise locked nucleotides, wherein the locked nucleotides may comprise locked adenine, locked cytosine, locked guanine, locked thymine and/or locked uracil. However, at least 0.06%, 0.07%, 0.08%, 0.09% or 0.10% of the constituent nucleotides in the resultant LNA-RNA molecule may comprise locked nucleotides, wherein the locked nucleotides may comprise locked adenine, locked cytosine, locked guanine, locked thymine and/or locked uracil. Preferably, at least 0.15%, 0.2%, 0.3%, 0.4% or 0.5% of the constituent nucleotides in the resultant LNA-RNA molecule may comprise locked nucleotides, wherein the locked nucleotides may comprise locked adenine, locked cytosine, locked guanine, locked thymine and/or locked uracil.
Preferably, at least 1%, 2% or 3% of the constituent nucleotides in the resultant RNA molecule are locked, wherein the locked nucleotides may comprise locked adenine, locked cytosine, locked guanine, locked thymine and/or locked uracil. More preferably, at least 4%, 5% or 6% of the constituent nucleotides in the resultant RNA molecule are locked, wherein the locked nucleotides may comprise locked adenine, locked cytosine, locked guanine, locked thymine and/or locked uracil. Even more preferably, at least 7%, 8% or 9% of the constituent nucleotides in the RNA molecule are locked, wherein the locked nucleotides may comprise locked adenine, locked cytosine, locked guanine, locked thymine and/or locked uracil. Still more preferably, at least 10%, 12% or 15% of the constituent nucleotides in the RNA molecule are locked, wherein the locked nucleotides may comprise locked adenine, locked cytosine, locked guanine, locked thymine and/or locked uracil. Most preferably, at least 17%, 20%, 22%, 25%, 27%, 30% or 35% of the constituent in the RNA molecule are locked, wherein the locked nucleotides may comprise locked adenine, locked cytosine, locked guanine, locked thymine and/or locked uracil. The data described in the Examples suggest that combinations of locked nucleotides (LNP-NTPs) could provide additional advantages. For example, combined locked nucleotide substitutions were assessed in HEK293T cells that have impaired innate sensing pathways for nucleic acids, using firefly luciferase as a marker of protein expression (see Figure 10). Maximal enhancement (up to 12-fold at 24h) was seen with combined substitution of LNA-ATP+LNA-5meCTP+LNP-TTP at a 100ng dose (see Figure 10). These data suggest that combined locked nucleotides have a greater potential to enhance expression in the absence of innate recognition than individual locked nucleotide substitutions (see Figure 3). The inventors also assessed locked nucleotide combinations in HeLa cells that have functional innate sensing pathways. In these experiments, combined substitution of LNA-ATP+LNA-5meCTP+LNA-TTP at a 100ng dose provided the highest enhancement of expression demonstrating a >200 fold enhancement at 48 and 72h. This was higher than that seen with substitution of all four locked nucleotides. Further assessment of locked nucleotide combinations in THP1 monocytic cells with robust innate sensing pathways demonstrated an even greater level of enhancement (see Figure 12). In these experiments, combined substitution of LNA-ATP+LNA-5meCTP+LNA-TTP at a 100ng dose demonstrated 200-fold enhancement at 24h and up to 600-fold enhancement at 48h. A similar level of enhancement was seen with substitutions of all four NTPs. These data indicate that the
incorporation of combined locked substitutions has an even greater impact on both the magnitude and duration of expression in cells with intact innate sensing pathways, here demonstrated using a monocytic cell line. It was also observed that substitution of combined locked nucleotides (see Figure 13) had a greater impact than individual locked nucleotides with respect to the secretion profile of an indicative panel of innate cytokines in supernatants harvested from transfected THP-1 cells (see Figure 9). Here two, three and four locked nucleotide combinations were able to suppress MCP-1, MCP-2, MIP-1 alpha and MIP-1 beta and reduce expression of Gro-alpha, IFN- beta, IFN-gamma, IP10 and MC3, where substitution with all four locked nucleotides was the most effective at suppressing responses across all of the analytes tested (see Figure 12). Moreover, experiments in human skin explants and in vivo murine studies have shown that LNA modified RNA increased both the magnitude and duration of expression in ex-vivo human skin explants and also in mice, where expression of LNA modified saRNA was greater than mRNA. Additionally, these data indicate that LNA modification reduces the expression of cytokines and chemokines induced by mRNA and saRNA injection in vivo. For example, such cytokines and chemokines can include CRO-alpha, IFN-alpha 2a, IFN-beta, IFN-gamma, IP-10, MCP-1, MCP-2, MCP-3, MIP-1 alpha, and/or MIP-1 beta. As such, these data indicate that incorporation locked nucleotides, and in particular locked uracil (LNA-UTP), locked thymine (LNA-TTP), locked cytosine (LNA-CTP), locked 5-methyl cytosine (LNA-5meCTP), locked adenine (LNA-ATP) and locked guanine (LNA-GTP), enhances the magnitude and duration of mRNA expression in cells that have impaired and active innate RNA sensing that may suppress RNA translation and increase degradation. Accordingly, preferably the method comprises using a combination of two, three or four different locked nucleotides selected from a group consisting of: locked adenine, locked cytosine, locked guanine, locked thymine and locked uracil. Referring to formula [I], preferably the locked nucleotides comprise R
1 which is , R
2 which is a bond, R
3 is H, R
4 is H, X
1 is O, X
2 is absent and L is CH2.

The ratio between the two, three, four different locked nucleotides may be varied in the transcription reaction. Accordingly, the inventors have assessed various different combinations of locked nucleotides (LNA-NTPs), as follows: For example, for mRNA these are: 1.75% LNA-ATP+90%LNA-5meCTP 2.90% LNA-ATP+90%LNA-CTP 3.90% LNA-ATP+75% LNA-GTP 4.90% LNA-ATP+90% LNA-TTP 5.90% LNA-ATP+90% LNA-UTP 6.90% LNA-5meCTP+75% LNA-GTP 7.90% LNA-CTP+75% LNA-GTP 8.90% LNA-5meCTP+90% LNA-TTP 9.90% LNA-CTP+90% LNA-TTP 10.90% LNA-5meCTP+90% LNA-UTP 11.90% LNA-CTP+90% LNA-UTP 12.75% LNA-GTP+90% LNA-TTP 13.75% LNA-GTP+90% LNA-UTP 14.90% LNA-ATP+90% LNA-5meCTP+75%LNA-GTP 15.90% LNA-ATP+90% LNA-CTP+75%LNA-GTP 16.75% LNA-ATP+90% LNA-5meCTP+90% LNA-TTP 17.75% LNA-ATP+90% LNA-CTP+90% LNA-TTP 18.75% LNA-ATP+90% LNA-5meCTP+90% LNA-UTP 19.75% LNA-ATP+90% LNA-CTP+90% LNA-UTP 20.75% LNA-ATP+75% LNA-GTP+90% LNA-TTP 2175% LNA-ATP+75% LNA-GTP+90% LNA-UTP 22.90% LNA-5meCTP+75% LNA-GTP+90% LNA-TTP 23.90% LNA-CTP+75% LNA-GTP+90% LNA-TTP 24.90% LNA-5meCTP+75% LNA-GTP+90% LNA-UTP 25.90% LNA-CTP+75% LNA-GTP+90% LNA-UTP 26.75% LNA-ATP+90% LNA-5meCTP+75% LNA-GTP+90% LNA-TTP 27.75% LNA-ATP+90% LNA-CTP+75% LNA-GTP+90% LNA-TTP 28.75% LNA-ATP+90% LNA-5meCTP+75% LNA-GTP+90% LNA-UTP 29.75% LNA-ATP+90% LNA-CTP+75% LNA-GTP+90% LNA-UTP; or any other percentage ratio.
For example, for saRNA these are: 1.75% LNA-ATP+90%LNA-5meCTP 2.90% LNA-ATP+90%LNA-CTP 3.90% LNA-ATP+75% LNA-GTP 4.90% LNA-ATP+90% LNA-TTP 5.90% LNA-ATP+90% LNA-UTP 6.90% LNA-5meCTP+75% LNA-GTP 7.90% LNA-CTP+75% LNA-GTP 8.90% LNA-5meCTP+90% LNA-TTP 9.90% LNA-CTP+90% LNA-TTP 10.90% LNA-5meCTP+90% LNA-UTP 11.90% LNA-CTP+90% LNA-UTP 12.75% LNA-GTP+90% LNA-TTP 13.75% LNA-GTP+90% LNA-UTP 14.90% LNA-ATP+90% LNA-5meCTP+75%LNA-GTP 15.90% LNA-ATP+90% LNA-CTP+75%LNA-GTP 16.75% LNA-ATP+90% LNA-5meCTP+90% LNA-TTP 17.75% LNA-ATP+90% LNA-CTP+90% LNA-TTP 18.90% LNA-ATP+90% LNA-CTP+90% LNA-TTP 19.75% LNA-ATP+90% LNA-5meCTP+90% LNA-UTP 20.75% LNA-ATP+90% LNA-CTP+90% LNA-UTP 21.90% LNA-ATP+90% LNA-CTP+90% LNA-UTP 22.75% LNA-ATP+75% LNA-GTP+90% LNA-TTP 2375% LNA-ATP+75% LNA-GTP+90% LNA-UTP 24.90% LNA-5meCTP+75% LNA-GTP+90% LNA-TTP 25.90% LNA-CTP+75% LNA-GTP+90% LNA-TTP 26.90% LNA-5meCTP+75% LNA-GTP+90% LNA-UTP 27.90% LNA-CTP+75% LNA-GTP+90% LNA-UTP 28.75% LNA-ATP+90% LNA-5meCTP+75% LNA-GTP+90% LNA-TTP 29.75% LNA-ATP+90% LNA-CTP+75% LNA-GTP+90% LNA-TTP 30.75% LNA-ATP+90% LNA-5meCTP+75% LNA-GTP+90% LNA-UTP 31.75% LNA-ATP+90% LNA-CTP+75% LNA-GTP+90% LNA-UTP; or any other percentage ratio. In preferred embodiments, the percentage ratio may be up to 100% substitution.
The RNA molecule may further or more modified nucleotide comprising a 2’-substituted group in which the OH group normally at the 2’ position is replaced with a halogen (preferably fluorine), an optionally substituted aromatic group, a NH
2, a N3, a H, an optionally substituted O-alkyl, O-alkenyl or O-alkynyl group, or an optionally substituted alkyl, alkenyl or alkynyl group, wherein in each instance the aromatic group, alkyl, alkenyl or alkynyl is optionally substituted with halogen, oxo, OR, CN, NR2 or SR, wherein R is H or C1-6 alkyl, C2-6 alkenyl or C2-6 alkynyl. Such a combination, in the RNA molecule, of one or more locked nucleotide (LNA) and one or more 2’-modified nucleotide can be referred to as a miximer. The 2’-modified nucleotide may be as defined in PCT/GB2023/050508, the contents of which are incorporated herein by reference. The one or more modified nucleotide may be represented herein by formula [II]:
[II] wherein Base is a nucleobase; one of R
6 and R
8 is a phosphate linker, a modified phosphate linker or a 5 to 10 membered heteroarylene, wherein the phosphate linker, the modified phosphate linker or the 5 to 10 membered heteroarylene is a linker between the rest of the locked nucleotide of formula [I] and an attachment point to a first adjacent nucleotide in the RNA molecule, and the other of R
6 and R
8 is a bond to a second adjacent nucleotide in the RNA molecule; R
7 and R
10 are independently H or C1-6 alkyl; R
9 is a halogen (preferably fluorine), an optionally substituted aromatic group, a NH
2, a N3, a H, an optionally substituted O-alkyl, O-alkenyl or O-alkynyl group, or an optionally substituted alkyl, alkenyl or alkynyl group, wherein in each instance the aromatic group, alkyl, alkenyl or alkynyl is optionally substituted with halogen, oxo, OR
11, CN, NR
11R
12 or SR
11; and R
11 and R
12 are independently H or C1-6 alkyl, C2-6 alkenyl or C2-6 alkynyl. The one or more modified nucleotide may be represented herein by formula [IIa]:
a]
The nucleobase may be as defined above in relation to formula [I]. One of R
6 and R
8 may be a phosphate linker or a modified phosphate linker. The phosphate linker or modified phosphate linker may be as defined above in relation to the definition of R
1 and R
2. Preferably, one of R
6 or R
8 and R
8 is a bond.
R
7 and R
10 may independently be H or C
1-3 alkyl, and more preferably are independently H or methyl. In some embodiments, R
7 and R
10 are both H. In one embodiment, R
9 is an optionally substituted O-alkyl, an optionally substituted O-alkenyl, an optionally substituted O-alkynyl group, an optionally substituted alkyl, an optionally substituted alkenyl or an optionally substituted alkynyl. It may be appreciated, when discussing the optional substituents and the size of the alkyl, alkenyl and/or alkynyl, this applies to both the optionally substituted alkyl, alkenyl and alkynyl groups per se as well as the optionally substituted O-alkyl, O- alkenyl and O-alkynyl groups. The alkyl, alkenyl or alkynyl may be an optionally substituted C1-C20 alkyl, alkenyl or alkynyl, wherein the alkyl, alkenyl or alkynyl is optionally substituted with halogen, oxo, OR
11, CN, NR
11R
12 or SR
11, wherein R
11 and R
12 are independently H or C1-6 alkyl, C2- 6 alkenyl or C2-6 alkynyl. Preferably, the alkyl, alkenyl or alkynyl may be an optionally substituted C
1-C
10 alkyl, alkenyl or alkynyl, wherein the alkyl, alkenyl or alkynyl is optionally substituted with halogen, oxo, OR
11, CN, NR
11R
12 or SR
11, wherein R
11 and R
12 are H or C1-6 alkyl, C2-6 alkenyl or C2-6 alkynyl.
In some embodiments, the alkyl, may be an optionally substituted C1- 6 alkyl, a C2-6 alkenyl or a C2-6 alkynyl, wherein the, or each alkyl, alkenyl or alkynyl is optionally substituted with halogen, oxo, OR
11, CN, NR
11R
12 or SR
11, wherein R
11 and R
12 are H or C1-6 alkyl, C2-6 alkenyl or C2-6 alkynyl. In some embodiments, the alkyl, alkenyl or alkynyl may be an optionally substituted C1-3 alkyl, a C2-3 alkenyl or a C2-3 alkynyl, wherein the, or each alkyl, alkenyl or alkynyl is substituted with one or more substituents selected from the group consisting of an oxo, OH, OMe, NH2 and NR
11H, wherein R
11 is C
1-6 alkyl, C
2-6 alkenyl or C
2-6 alkynyl. More preferably, the alkyl, alkenyl or alkynyl may be an optionally substituted C1-3 alkyl, a C2-3 alkenyl or a C2-3 alkynyl, wherein the, or each alkyl, alkenyl or alkynyl is preferably unsubstituted. Accordingly, in some embodiments, the alkyl, alkenyl or alkynyl may be methyl, ethyl, propyl, ethenyl, propenyl, ethynyl or propynyl, optionally substituted with one or more substituents selected from the group consisting of an oxo, OH, OMe, NH2 and NR
11H, wherein R
11 is C1-6 alkyl, C2-6 alkenyl or C2-6 alkynyl. Accordingly, the alkyl, alkenyl or alkynyl may be methyl, hydromethyl, acetyl, formyl, carbamoylmethyl, methoxycarbonylmethyl, carboxyhydroxymethyl, carboxymethylaminomethyl, oxyacetic acid methyl ester or isopentenylaminomethyl. Preferably, R
9 is a halogen. The halogen may be a chlorine, or bromine. Preferably the halogen is fluorine. Therefore, the one or more modified nucleotide preferably comprises a 2’-substituted fluoro nucleotide. R
9 may be an optionally substituted aromatic group. The optionally substituted aromatic group may be a C6-C12 optionally substituted aromatic group. The optionally substituted aromatic group may be optionally substituted phenyl. Preferably, R
9 is Me or OMe, more preferably OMe. Accordingly, preferably the use or method comprises using at least one modified nucleotide selected from a group consisting of: a modified adenine; a modified cytosine; a modified guanine; a modified uracil and/or a modified thymine. Preferably, the use or method comprises using at least two modified nucleotides selected from a group consisting of: a modified adenine; a modified cytosine; a modified guanine; a modified uracil and/or a modified thymine. Preferably, the use or method comprises using at least three modified nucleotides selected from a group consisting of: a
modified adenine; a modified cytosine; guanine; a modified uracil and/or a modified thymine. Preferably, the use or method comprises using at least four modified nucleotides selected from a group consisting of: a modified adenine; a modified cytosine; a modified guanine; a modified uracil and/or a modified thymine. Preferably, the use or method comprises using at least five modified nucleotides selected from a group consisting of: a modified adenine; a modified cytosine; a modified guanine; a modified uracil and/or a modified thymine. Preferably, the one or more modified nucleotide comprises a 2’-methyl modified nucleotide. Most preferably, the one or more modified nucleotide comprises a 2’-O-methyl modified nucleotide. There are at least 28 identified natural 2’-O-methyl modifications (Nm) to ribonucleosides. Accordingly, the one or more modified nucleotide may be selected from a group consisting of: 2’-O-methyladenosine (Am); 1,2’-dimethyl-adenosine (m
1Am); N6,2’-O-dimethyl-adenosine (m
6Am); N
6,N
6,2-O-trimethyl-adenosine (m
6,6Am); 2’-O-methylinosine (Im); 1,2’-O-dimethylinosine (m
1Im); 2’-O- methylguanosine (Gm); 1,2’-O-dimethylguanosine (m
1Gm); N2,2’-O- dimethylguanosine (m
2Gm); N2,N2, 2’-O-trimethylguanosine (m
2,2Gm); N2,7,2'-O- trimethyl-guanosine (m
2,7Gm); 2’-O-methylcytidine; N
4,2’-O-dimethyl cytidine(m
4Cm); N
4,N
4,2-O-trimethyl-cytidine (m
4,4Cm); 5,2’-O-dimethyl-cytidine (m
5Cm); N
4-acetyl-2’- O-methyl-cytidine (ac
4Cm); 2’-O-methyl-5-hydromethyl-cytidine (hm
5Cm); 5-formyl- 2’-O-methyl-cytidine (f
5Cm); 2’-O-methyluridine (Um); 3,2’-O-dimethyluridine (m
3Um); 5,2’-O-dimethyluridine (m
5Um or Tm); 2-thio-2’-O-methyl-uridine (s
2Um); 2’-O-methyl-pseudouridine (Ym); 5-carbamoylmethyl-2’-O-methyluridine (ncm
5Um); 5-methoxycarbonylmethyl-2’-O-methyluridine (mcm
5Um); 5-(carboxyhydroxymethyl)- 2’-O-methyluridine methyl ester (mchm
5Um); 5-carboxymethylaminomethyl-2’-O- methyluridine (cmbm
5Um); 2’-O-methyluridine 5-oxyacetic acid methyl ester (mcmo
5Um); 5-(isopentenylaminomethyl)-3’O-methyluridine (inm
5Um); 2’-O- (trifluoromethyl)adenosine, 2’-O-(trifluoromethyl)guanosine, 2’-O- (trifluoromethyl)cytosine, 2’-O-(trifluoromethyl)uridine; 2’-O- (trifluoromethyl)thymidine; and any other synthetic nucleotide or NTP that contains a 2’-O-methylated ribose.
Preferably, therefore, the one or more nucleotide comprises a 2’-O-methyl modified adenine (2’-O-methyl-Adenine; also known as “2-OMe ATP” or “Am”), a 2’-O- methyl modified cytosine (2’-O-methyl-Cytosine; also known as “2-OMe CTP” or “Cm”), a 2’-O-methyl modified guanine (2’-O-methyl-Guanine; also known as “2-OMe GTP” or “Gm”), a 2’-O-methyl modified uracil (2’-O-methyl-Uracil; also known as “2- OMe UTP” or “Um”), and/or a 2’-O-methyl modified thymine (2’-O-methyl-Thymine; also known as “2-OMe TTP” or “Tm”). Preferably, the method comprises using one or more modified nucleotide which comprises a 2’-O-methyl-ATP, a 2’-O-methyl-CTP, a 2’-O-methyl-GTP, a 2’-O-methyl- UTP, and/or 2’-O-methyl-TTP. Preferably, the method comprises using at least one modified nucleotide selected from a group consisting of: 2’-O-methyl-ATP, a 2’-O- methyl-CTP, a 2’-O-methyl-GTP, a 2’-O-methyl-UTP, and 2’-O-methyl-TTP. Preferably, the method comprises using at least two modified nucleotides selected from a group consisting of: 2’-O-methyl-ATP, a 2’-O-methyl-CTP, a 2’-O-methyl-GTP, a 2’-O- methyl-UTP, and 2’-O-methyl-TTP. Preferably, the method comprises using at least three modified nucleotides selected from a group consisting of: 2’-O-methyl-ATP, a 2’- O-methyl-CTP, a 2’-O-methyl-GTP, a 2’-O-methyl-UTP, and 2’-O-methyl-TTP. Preferably, the method comprises using at least four modified nucleotides selected from a group consisting of: 2’-O-methyl-ATP, a 2’-O-methyl-CTP, a 2’-O-methyl-GTP, a 2’-O- methyl-UTP, and 2’-O-methyl-TTP. Preferably, the method comprises using at least five modified nucleotides selected from a group consisting of: 2’-O-methyl-ATP, a 2’-O- methyl-CTP, a 2’-O-methyl-GTP, a 2’-O-methyl-UTP, and 2’-O-methyl-TTP. Preferably, the method comprises using at least four modified nucleotides selected from a group consisting of: 2’-O-methyl-ATP, a 2’-O-methyl-CTP, a 2’-O-methyl-GTP, and a 2’-O-methyl-UTP. Most preferably, however, the method comprises using a modified nucleotide which comprises a 2’-substituted fluoro nucleotide. In a preferred embodiment, the use or method of the invention comprises using a combination of: (i) one or more locked nucleotide represented by formula [I]; and (ii) one or more modified nucleotide represented by formula [II].
Preferably, the use or method of the comprises using a combination of: (i) one or more locked nucleotide represented by formula [I], wherein R
1 is a bond, R
3 is H, R
4 is H, X
1 is O, X
2 is absent and L is
adenine, cytosine, 5-methyl-cytosine, guanine, uracil or (ii) one or more modified nucleotide represented by formula [II], wherein R
6 is , R
7 is hydrogen, R
8 is a bond, R
9 is a halogen (preferably fluorine), R
10 is hydrogen, and the base is adenine, cytosine, 5-methyl- cytosine, guanine, uracil or thymine. The method may further comprise use of DTT. This is thought to enhance RNA yield and RNA polymerase activity. The method may further comprise use of a pH buffer, such as HEPES, though the skilled person would appreciate that other buffers are available. The method may further comprise the use of a crowding agent, for example Poly(ethylene glycol) (PEG) of various molecular weights. For example, the crowding agent may be PEG200 or any additional crowding agent [32]. The method may further comprise use of an RNase inhibitor. This prevents RNA degradation if any RNase is present in the reaction. The method may further comprise use of spermidine. This is thought to improve the activity of the RNA polymerase and therefore the RNA yield. The template nucleic acid sequence is preferably transcribed by the RNA polymerase to produce the modified RNA molecule. Preferably, therefore, the template nucleic acid sequence comprises DNA. The template nucleic acid may be made synthetically, for example doggybone DNA, or by PCR, rolling circle amplification, or synthetic amplification. The template nucleic acid may comprise a vector, and is preferably a plasmid.
In one embodiment, the LNA-RNA molecule may be used in the manufacture of recombinant proteins, for example in a cell free system. In a further embodiement the LNA-RNA may be used in the manufacture of cultured meat. In another embodiment the LNA-RNA molecule may be used to differentiate stem cells, preferably ex vivo. In one embodiment, the LNA-RNA molecule may also be used in the manufacture of RNAi-based biopesticides against viral and fungal infection/disease/spoilage. In addition, LNA-RNA may be used against pests, such as insects (arthropods). Degradation of RNAi in the environment can occur via the actions of ribonucleases, here the use of LNA-RNA with enhanced resistance against ribonucleases is anticipated by the inventors to provide significant advantages for the generation of effective RNAi based biopesticides. In one embodiment, the template nucleic acid may be transcribed to create the resultant modified RNA molecule, which can be described as being a biotherapeutic RNA molecule, i.e. one which is used therapeutically to treat, prevent or ameliorate a disease in a patient. The template nucleic acid sequence may encode a therapeutic protein, which is derived from an animal or a human, and which treats, prevents or ameliorates disease in a subject, preferably a human or animal subject. For example, therapeutic uses of the encoded therapeutic protein may include protein replacement, gene editing (e.g. CRISPR-Cas9), gene therapy, or RNAi, and so on. The biotherapeutic RNA molecule may be selected from a group consisting of: messenger RNA (mRNA), micro RNA (miRNA); short interfering RNA (siRNA); short hairpin RNA (shRNA); anti-sense RNA; RNA aptamer; self-amplifying RNA (saRNA); interference RNA (RNAi); non-coding RNA; circular RNA; and small RNA. In one embodiment, the template nucleic acid may encode a protein and peptide derived from a plant. Preferably, the protein and peptide is a plant antigen. The plant antigen may be derived from Ricinus spp., preferably Ricinus communis. It will be appreciated that RNAi may be used as an insecticide. Thus, the LNA-RNA molecule may be used as an insecticide.
In another embodiment, the template acid encodes an antigen, in which case the resultant modified RNA molecule can be described as being a vaccine, i.e. one which is used prophylactically to prevent a disease in a patient, or one which is used therapeutically to ameliorate disease, such as cancer. For example, the template nucleic acid sequence may encode an antigen which is derived from a virus, a bacteria, a mycoplasma, a fungus, an animal, a plant, an alga, a parasite, or a protozoan, or other organism which causes a disease in a subject, preferably a human or animal. Preferably, the template nucleic acid sequence is from a virus or a bacterium. For example, the template nucleic acid may encode at least a portion of a viral spike protein. In another embodiment, the template nucleic acid may encode a tumour immunogen or antigen, or cancer immunogen or antigen. The tumour immunogen and antigen may be a peptide-containing tumour antigen, such as a polypeptide tumour antigen or glycoprotein tumour antigens. The tumour antigen may be (a) a full length molecule associated with cancer cells, (b) a homolog and modified form of the same, including molecules with deleted, added and/or substituted portions, and (c) a fragment of the same including peptides or strings of peptides. Suitable tumour immunogens include: class I-restricted antigens recognized by CD 8+ lymphocytes or class II-restricted antigens recognized by CD4+ lymphocytes. The tumour antigen may be an antigen that is associated with a cancer selected from the group consisting of: a testis cancer, melanoma, lung cancer, head and neck cancer, NSCLC, breast cancer, gastrointestinal cancer, bladder cancer, colorectal cancer, pancreatic cancer, lymphoma, leukaemia, renal cancer, hepatoma, ovarian cancer, gastric cancer and prostate cancer. The tumour antigen may be selected from: (a) cancer-testis antigens such as NY-ESO-I, SSX2, SCPl as well as RAGE, BAGE, GAGE and MAGE family polypeptides, for example, GAGE-I, GAGE-2, MAGE-I, MAGE-2, MAGE-3, MAGE-4, MAGE-5, MAGE-6, and MAGE- 12 (which can be used, for example, to address melanoma, lung, head and neck, NSCLC, breast, gastrointestinal, and bladder tumours);
(b) mutated antigens, for example, p53 with various solid tumours, e.g., colorectal, lung, head and neck cancer), p21/Ras (associated with, e.g., melanoma, pancreatic cancer and colorectal cancer), CDK4 (associated with, e.g., melanoma), MUMl (associated with, e.g., melanoma), caspase-8 (associated with, e.g., head and neck cancer), CIA 0205 (associated with, e.g., bladder cancer), HLA-A2-R1701, beta catenin (associated with, e.g., melanoma), TCR (associated with, e.g., T- cell non-Hodgkins lymphoma), BCR- abl (associated with, e.g., chronic myelogenous leukaemia), triosephosphate isomerase, KIA 0205, CDC-27, and LDLR-FUT; (c) over-expressed antigens, for example, Galectin 4 (associated with, e.g., colorectal cancer), Galectin 9 (associated with, e.g., Hodgkin's disease), proteinase 3 (associated with, e.g., chronic myelogenous leukaemia), WT 1 (associated with, e.g., various leukaemias), carbonic anhydrase (associated with, e.g., renal cancer), aldolase A (associated with, e.g., lung cancer), PRAME (associated with, e.g., melanoma), HER- 2/neu (associated with, e.g., breast, colon, lung and ovarian cancer), alpha- fetoprotein (associated with, e.g., hepatoma), KSA (associated with, e.g., colorectal cancer), gastrin (associated with, e.g., pancreatic and gastric cancer), telomerase catalytic protein, MUC-I (associated with, e.g., breast and ovarian cancer), G-250 (associated with, e.g., renal cell carcinoma), p53 (associated with, e.g., breast, colon cancer), and carcinoembryonic antigen (associated with, e.g., breast cancer, lung cancer, and cancers of the gastrointestinal tract such as colorectal cancer); (d) shared antigens, for example, melanoma-melanocyte differentiation antigens such as MART-1/Melan A, gplOO, MClR, melanocyte-stimulating hormone receptor, tyrosinase, tyrosinase related protein- 1 /TRPl and tyrosinase related protein-2/TRP2 (associated with, e.g., melanoma); (e) prostate-associated antigens, such as PAP, PSA, PSMA, PSH-Pl, PSM-Pl, PSM-P2, associated with e.g., prostate cancer; and/or (f) immunoglobulin idiotypes (associated with myeloma and B cell lymphomas, for example). The template nucleic acid may encode a eukaryotic polypeptide. In one embodiment the eukaryotic polypeptide is a mammalian polypeptide. The mammalian polypeptide may be selected from the group consisting of: an enzyme; an enzyme inhibitor; a hormone;
an immune system protein; a receptor; protein; a transcription or translation factor; tumour growth supressing protein; a structural protein and a blood protein. The enzyme may be selected from the group consisting of: chymosin; gastric lipase; tissue plasminogen activator; streptokinase; a cholesterol biosynthetic or degradative steriodogenic enzyme; kinases; phosphodiesterases; methylases; de-methylases; dehydrogenases; cellulases; proteases; lipases; phospholipases; aromatases; cytochromes; adenylate or guanylaste cyclases and neuramidases. The enzyme inhibitor may be tissue inhibitor of metalloproteinase (TIMP). The hormone may be growth hormone. The immune system protein may be selected from the group consisting of: a cytokine; a chemokine; a lymphokine; erythropoietin; an integrin; addressin; selectin; homing receptors; T cell receptors and immunoglobulins. The cytokine may be an interleukin, for example IL-2, IL-4 and/or IL-6, colony stimulating factor (CSF), granulocyte colony stimulating factor (G- CSF), granulocyte- macrophage colony stimulating factor (GM-CSF) or tumour necrosis factor (TNF). The chemokine may be a macrophage inflammatory protein-2 and/or a plasminogen activator. The lymphokine may be an interferon. The immunoglobulin may be a natural, modified or chimeric immunoglobulin or a fragment thereof. Preferably, the immunoglobulin is a chimeric immunoglobulin having dual activity such as antibody enzyme or antibody-toxin chimera. The hormone may be selected from the group consisting of: insulin, thyroid hormone, catecholamines, gonadotrophines, trophic hormones, prolactin, oxytocin, dopamine, bovine somatotropin, leptins; growth hormones (e.g., human grown hormone), growth factors (e.g., epidermal growth factor, nerve growth factor, insulin-like growth factor and the like).
The receptor may be a steroid hormone or a peptide receptor. Preferably, the receptor is a growth factor receptor. The binding protein may be a growth factor binding protein. The tumour growth suppressing protein may be a protein that inhibits angiogenesis. The structural protein may be selected from the group consisting of: collagen; fibroin; fibrinogen; elastin; tubulin; actin; and myosin. The blood protein may be selected from the group consisting of thrombin; serum albumin; Factor VII; Factor VIII; insulin; Factor IX; Factor X; tissue plasminogen activator; protein C; von Willebrand factor; antithrombin III; glucocerebrosidase; erythropoietin granulocyte colony stimulating factor (GCSF) or modified Factor VIII; and anticoagulants. In one preferred embodiment, the template nucleic acid may encode a cytokine which is capable of regulating lymphoid homeostasis, preferably a cytokine which is involved in and preferably induces or enhances development, priming, expansion, differentiation and/or survival of T cells. Thus, preferably, the cytokine is an interleukin. Most preferably, IL-2, IL-7, IL-12, IL-15, or IL-21. The template nucleic acid may encode a protein that is capable of enhancing reprogramming of somatic cells to cells having stem cell characteristics. The protein that is capable of enhancing reprogramming of somatic cells to cells having stem cell characteristics may be selected from the group consisting of: OCT4, SOX2, NANOG, LIN28, p53, ART-4, BAGE, ss- catenin/m, Bcr-abL CAMEL, CAP-1, CASP-8, CDC27/m, CD 4/m, CEA, CLAUDIN-12, c- MYC, CT, Cyp-B, DAM, ELF2M, ETV6- AML1, G250, GAGE, GnT-V, GaplOO, HAGE, HER-2/neu, HPV-E7, HPV-E6, HAST-2, hTERT (or hTRT), LAGE, LDLR/FUT, MAGE-A, MAGE-B, MAGE- C, MART- 1/Melan- A, MC1R, Myosin/m, MUC1, MUM-1, -2, -3, NA88-A, NF1, NY-ESO- 1, NY-BR-1, pl90 minor BCR-abL, Plac-1, Pml/RARa, PRAME, proteinase 3, PSA, PSM, RAGE, RU1 or RU2, SAGE, SART-1 or SART-3, SCGB3A2, SCP1, SCP2, SCP3, SSX, SURVIVIN, TEL/AML1, TPI/m, TRP-1, TRP-2, TRP-2/INT2, TPTE and WT, preferably WT-1.
Preferably, MAGE-A is selected from consisting of: MAGE-A 1, MAGE-A2, MAGE-A3, MAGE-A4, MAGE-A5, MAGE-A6, MAGE- A7, MAGE-A8, MAGE-A9, MAGE-A 10, MAGE-A 11, or MAGE-A 12 Preferably, the protein that is capable of enhancing reprogramming of somatic cells to cells having stem cell characteristics is OCT4, SOX2, LF4; c-MYC; NANOG; LIN28. The template nucleic acid may encode a biomolecule that is utilised for the modification of cells ex vivo for cell-therapy indications. Thus, preferably the template nucleic acid may encode a protein selected from the group consisting of an immunoglobulin, a T- cell receptor and NK receptor. The template nucleic acid may encode a protein that is capable of regulating expression of endogenous host genes, for example an interfering RNA, such as small RNAs, siRNA or microRNAs. In an embodiment, the template nucleic acid may encode an innate inhibitor protein, as described in WO2020/254804, PCT/GB2021/053362 or PCT/GB2021/053361, which counteracts the innate immune response in a subject administered with a vaccine comprising the resultant RNA molecule. In accordance with an eighth aspect, there is provided an RNA molecule obtained or obtainable by the method according to the sixth aspect. In a ninth aspect, there is provided an RNA molecule comprising at least 25 nucleotides, wherein one or more of the nucleotides is a locked nucleotide. The RNA molecule of the ninth aspect may be defined as in any of the previous aspects. For example, the type and length of the RNA molecule may be as described herein. Also, the type and amount of locked nucleotide incorporated in the RNA molecule may be as described herein. In a tenth aspect, there is provided a pharmaceutical composition comprising the RNA molecule according to the eighth or ninth aspect and a pharmaceutically acceptable vehicle.
In an eleventh aspect, there is provided of preparing the pharmaceutical composition according to the tenth aspect, the method comprising contacting the RNA molecule according to the eighth or ninth aspect and a pharmaceutically acceptable vehicle. In a twelfth aspect, there is provided the RNA molecule according to the eighth or ninth aspect, or the pharmaceutical composition according to the tenth aspect, for use as a medicament. In a thirteenth aspect, there is provided the RNA molecule according to the eighth or ninth aspect, or the pharmaceutical composition according to the tenth aspect, for use in treating, preventing or ameliorating a disease in a subject, such as cancer. In a fourteenth aspect, there is provided a method of treating, preventing or ameliorating a disease in a subject, such as cancer, the method comprising administering, or having administered, to a subject in need thereof, a therapeutically effective amount of the RNA molecule according to the eighth or ninth aspect, or the pharmaceutical composition according to the tenth aspect. In a fifteenth aspect, there is provided a vaccine composition comprising the RNA molecule according to the eighth or ninth aspect, or the pharmaceutical composition according to the tenth aspect. The vaccine may comprise a suitable adjuvant. Examples of adjuvants may include an aluminium salt, a synthetic form of DNA, a carbohydrate, a tablet binder, an ion exchange resin, a preservative, a polymer, an emulsion and/or a lipid. Examples of adjuvants may include monosodium glutamate, sucrose, dextrose, aluminum bovine, human serum albumin, cytosine phosphoguanine, potassium phosphate, plasdone C, anhydrous lactose, cellulose, polacrilin potassium, glycerine, asparagine, citric acid, potassium phosphate magnesium sulfate, iron ammonium citrate, 2-phenoxyethanol, aluminium, beta-propiolactone, bovine extract, DOPC, EDTA, formaldehyde, Cytosine phosphoguanine (CpG), QS21, saponin, Monophosphoryl lipid A (MPLA), squalene, thimerosal, phenol, potassium aluminum sulfate, potassium glutamate, sodium borate, sodium metabisulphite, urea, PLGA, PVA, PLA, PVP, cyclodextrin-based stabilisers, oil in water emulsion adjuvants and/or lipid-based adjuvants.
In a sixteenth aspect, there is provided molecule according to the eighth or ninth aspect, the pharmaceutical composition according to the tenth aspect or the vaccine according to the fifteenth aspect, for use in stimulating an immune response in a subject. The immune response may be stimulated against a protozoa, bacterium, virus, fungus or cancer. Thus, the vaccine may be used to express a microbial or pathogenic antigen for immunizing against infectious micro-organisms, such as viruses and bacteria etc. However, the vaccine may also be used to express an oncogenic antigen, for preventing cancer. In a seventeenth aspect of the invention, there is provided a method of vaccinating a subject, the method comprising administering, or having administered, to a subject in need thereof, a therapeutically effective amount of the RNA molecule according to the eighth or ninth aspect, the pharmaceutical composition according to the tenth aspect or the vaccine according to the fifteenth aspect. It will be appreciated that RNA molecules can have significant utility in a wide range of therapeutic applications, for example RNAi, inhibitory RNA, RNA aptamers, and so on, in which translation of the RNA molecule does not necessarily need to occur. However, applications in which the RNA molecule does need to be translated include various vaccine and biotherapeutic approaches, such as the use of mRNA, saRNA, circular RNA, or any RNA sequence that is able to undergo translation and generate a peptide or protein in vivo. For example, the peptide or protein that is encoded by the RNA molecule may be involved in protein replacement, gene editing and gene therapy etc. It will also be appreciated that there is a growing trend for the use, in particular, of mRNA and saRNA vaccines, in which the RNA molecule encodes a pathogenic, such as a viral coat protein or region thereof, or an oncogenic antigen, and it is this antigen which is expressed in an immunised host organism and which triggers an immune response to the pathogen or the tumour. As described in the Examples, rather than directly measuring the amount of luciferase protein produced, the inventors have instead measured the enhancement of expression
in terms of luciferase activity. can, therefore, be considered as a surrogate of RNA translation, because it should directly relate to the amount of translation. Moreover, the luciferase data described in the examples can be used a surrogate of a therapeutic, essentially mirroring the expression of a therapeutic protein. However, as described herein, when the term “10-fold” or the like is used, it means that there is in fact a 10-fold increase in luciferase activity. It will be appreciated that the increase in expression or RNA translation may be related to the RNA being more stable, and so more protein is made before it is degraded. Accordingly, there may not be a change in translation efficiency (i.e. ribosomal speed), but an increase in the overall time that ribosomes have for making more protein. In summary, these data, when taken together, demonstrate that locked nucleotides can be used to increase the level of RNA translation for protein production in vitro and in vivo, the latter being especially advantageous when the RNA molecule is used as a vaccine or a therapeutic biomolecule. Based on the unexpected findings that incorporation locked nucleotides enhances, rather than reduces, expression, the inventors believe that their data support the finding that modified bases can be used to enhance the stability of RNA, as well as reduce the activation of innate sensing, interferon generation and/or degradation of an RNA molecule. Suitably, the one or more locked nucleotide enhances the level of expression and/or translation of the RNA molecule by at least 5- fold, 10-fold or 2-fold increase compared to the level of expression and/or translation that occurs in the absence of the one or more locked nucleotide. More suitably, the one or more locked nucleotide enhances the level of expression and/or translation of the RNA molecule by at least 5-fold, 10-fold or 50-fold compared to the level of expression and/or translation that occurs in the absence of the one or more locked nucleotide. Even more suitably, the one or more locked nucleotide enhances the level of expression and/or translation of the RNA molecule by at least 75-fold, 100-fold or 250-fold compared to the level of expression and/or translation that occurs in the absence of the one or more locked nucleotide. Preferably, the one or more locked nucleotide enhances the level of expression and/or translation of the RNA molecule by at least 500-fold, 750-fold or 1000-fold compared to the level of expression and/or translation that occurs in the absence of the one or more locked nucleotide. Preferably, the one or more locked nucleotide enhances the level of expression and/or translation of the RNA molecule by at least 2000-fold, 5000-
fold or 8000-fold increase compared to of expression and/or translation that occurs in the absence of the one or more locked nucleotide. The level of expression and/or translation may be measured by the expression of a fluorescent or luminescent protein encoded as a gene of interest in the RNA molecule (e.g. in either mRNA or saRNA). For example, the luminescent protein may be a bioluminescent protein, such as a luciferase (e.g. firefly luciferase protein encoded as a gene of interest). Thus, expression of luciferase can be determined by measurement of its enzymatic activity, where luciferin is converted to oxyluciferin emitting light that can be quantitated as relative light units (RLUs). The presence of light is proportional to the amount of translated protein. This approach was previously used to assess the impact of pseudouridine incorporation on translational efficiency [19]. The enzymatic incorporation of locked nucleotides in RNA has many potential outcomes and advantages selected from a group consisting of: changing mRNA stability; preventing innate recognition; reducing RNA degradation; increasing RNA half-life; enhancing recruitment of translational factors and/or ribosomes; and increasing translation. Preferably, the methods or uses enhance protein expression from mRNA, saRNA, circular RNA or any RNA sequence capable to being translated to generate a recombinant protein or peptide. In another embodiment, the methods or uses enhance the stability of RNA (be it mRNA, miRNA; siRNA; shRNA; anti-sense RNA; RNA aptamers; self-amplifying RNA (saRNA); interference RNA (RNAi); non-coding RNA; circular RNA; or small RNA). Improved stability provides a significant advantage to both coding and non-coding biotherapeutic or prophylatic uses of RNA molecules. The methods described herein allow the incorporation of locked nucleotides of any length (be they coding or non-coding) providing enhanced stability of the resultant RNA molecule. Stability of RNA molecules can be measured by quantitative PCR, but is also reflected by the duration of expression, where faster degradation equates to shorter expression.
In another embodiment, the methods result in reducing or eliminating innate sensing of RNA by membrane, endosomal and cellular RNA binding proteins that recognise unmodified RNA structures that trigger interferon signalling pathways and activation of cytoplasmic nucleases that degrade RNA such as RNase L [23]. Reduced innate sensing of RNA (be it mRNA, miRNA; siRNA; shRNA; anti-sense RNA; RNA aptamers; self-amplifying RNA (saRNA); interference RNA (RNAi); non-coding RNA; circular RNA; and small RNA) would provide significant advantages to both coding and non-coding biotherapeutic or prophylatic uses of RNA. The methods presented here allow incorporation of locked nucleotides of any length to prevent innate recognition of RNA, interferon induction and RNA degradation. The data described in the Examples show that incorporation of individual locked nucleotides surprisingly increases both the magnitude and expression of uncapped mRNA in cells that have impaired innate sensing mechanisms for recognizing RNA. Reducing the activation of innate sensing, leading to degradation of an RNA molecule is inferred by improved expression of modified saRNA and mRNA in interferon competent cells (HeLa and THP-1 cells), human skin explants and in vivo murine studies. Although the incorporation of locked nucleotides provides some improvement in expression in HEK293T cells, these cells have impaired innate sensing mechanisms, therefore, any improvement in expression directly relates to improved RNA half-life in the absence of innate recognition. Expression of unmodified saRNA and mRNA, if greatly diminished in both HeLa and THP-1 cells, reflects enhanced degradation caused by the triggering of innate sensing mechanisms. The incorporation of locked nucleotides in saRNA and mRNA provides more pronounced increase of expression in these two interferon-competent cell types (fold change) than that observed in HEK293T cells. Induction of type I interferon expression can be measured by ELISA as a surrogate of innate activation. It was observed that substitution of individual nucleotides with locked nucleotides was able to influence the secretion profile of a panel of cytokines in supernatants harvested from transfected THP-1 cells (Figure 9), in particular locked guanine was able to reduce IFN-alpha, locked adenine and locked uracil reduced IP-10 and all four locked nucleotides (ATP, CTP, GTP, UTP) reduced MCP1 and MCP2 with a hierarchy of GTP>UTP>CTP>ATP.
These data indicate that the locked nucleotides enhance both the magnitude and duration of expression in epithelial cells with intact innate sensing pathways (Figure 5 & 6), where the impact hierarchy on expression for the individual NTPs is UTP=TTP>CTP=5meCTP>ATP>GTP. Moreover, experiments in human skin explants and in vivo murine studies have shown that LNA modified RNA increased both the magnitude and duration of expression in ex-vivo human skin explants and also in mice, where expression of LNA modified saRNA was greater than mRNA. Additionally, these data indicate that LNA modification reduces the expression of cytokines and chemokines induced by mRNA and saRNA injection in vivo. For example, such cytokines and chemokines can include CRO-alpha, IFN-alpha 2a, IFN-beta, IFN-gamma, IP-10, MCP-1, MCP-2, MCP-3, MIP-1 alpha, and/or MIP-1 beta. It will be appreciated that the composition, the pharmaceutical composition, the vaccine or the therapeutic RNA molecule, may be used in a medicament, which may be used as a monotherapy (i.e. use of the composition alone). Alternatively, the composition according to the invention may be used as an adjunct to, or in combination with, known therapies for treating, ameliorating, or preventing an infection or disease. The composition of the invention may be combined in compositions having a number of different forms depending, in particular, on the manner in which the composition is to be used. Thus, for example, the composition may be in the form of a powder, tablet, capsule, liquid, ointment, cream, gel, hydrogel, aerosol, spray, micellar solution, transdermal patch, liposome suspension, polyplex, emulsion, liposome, lipid nanoparticles, functionalised liposomes or lipid nanoparticles (e.g. with peptide, antibody, antibody fragment, glycan, glyco-conjugate, DNA or RNA on the surface or encapsulated) or any other suitable form that may be administered to a person or animal. The lipid nanoparticle may comprise one or more components selected from a group consisting of: a cationic lipid (which is preferably ionisable); phosphatidylcholine; cholesterol; and polyethylene glycol (PEG)-lipid. It will be appreciated that the vehicle of medicaments according to the invention should be one which is well-tolerated by the subject to whom it is given. Medicaments comprising the composition, the pharmaceutical composition or the vaccine of the invention may be used in a number of ways. For instance, oral
administration may be required, in the agents may be contained within a composition that may, for example, be ingested orally in the form of a tablet, capsule or liquid. Compositions comprising agents and medicaments of the invention may be administered by inhalation (e.g. intranasally). Compositions may also be formulated for topical use. For instance, creams or ointments may be applied to the skin. Formulations may be provided in a lyophilised format for reconstitution or as a solid dosage form for topical, inserted or injected delivery. The composition, the pharmaceutical composition, vaccine or therapeutic RNA molecule of the invention may also be incorporated within a slow- or delayed-release device. Such devices may, for example, be inserted on or under the skin, and the medicament may be released over weeks or even months. The device may be located at least adjacent the treatment site. In a preferred embodiment, however, medicaments according to the invention may be administered to a subject by injection into the blood stream, muscle, skin or directly into a site requiring treatment. Injections may be intravenous (bolus or infusion), subcutaneous (bolus or infusion), intradermal (bolus or infusion), intramuscular (bolus or infusion), intrathecal (bolus or infusion), epidural (bolus or infusion) or intraperitoneal (bolus or infusion). It will be appreciated that the amount of composition, the pharmaceutical composition or the vaccine or therapeutic RNA molecule that is required is determined by its biological activity and bioavailability, which in turn depends on the mode of administration, the physiochemical properties of the composition, the pharmaceutical composition or the vaccine and whether it is being used as a monotherapy or in a combined therapy. The frequency of administration will also be influenced by the half-life of the active agent within the subject being treated. Optimal dosages to be administered may be determined by those skilled in the art, and will vary with the composition, the pharmaceutical composition or the vaccine in use, the strength of the pharmaceutical composition, the mode of administration, and the type of treatment. Additional factors depending on the particular subject being treated will result in a need to adjust dosages, including subject age, weight, gender, diet, and time of administration.
The required dose may depend upon a of factors including, but not limited to, the active agent being administered, the disease being treated and/or vaccinated against, the subject being treated, etc. Generally, a dose of between 0.001 µg/kg of body weight and 10 mg/kg of body weight, or between 0.01 ^g/kg of body weight and 1 mg/kg of body weight, of the composition, the pharmaceutical composition or the vaccine or therapeutic RNA molecule of the invention may be used, depending upon the active agent used. A dose may be understood to relate to the quantity of the RNA molecule which is delivered. Doses may be given as a single administration (e.g., a single injection). Alternatively, the composition, the pharmaceutical composition, biotherapeutic RNA molecule or the vaccine may require more than one administration. As an example, the composition, the pharmaceutical composition or the vaccine or therapeutic RNA molecule may be administered as an initial primer and a subsequent boost(s), or two boosts administered at between a week or monthly intervals (e.g. two or more doses of between 0.07 ^g and 700 mg, i.e., assuming a body weight of 70 kg), or for a biotherapeutic repeat dosing on a daily, weekly or monthly basis. Alternatively, a slow- release device may be used to provide optimal doses of the composition, the pharmaceutical composition or the vaccine or therapeutic RNA molecule according to the invention to a patient without the need to administer repeated doses. Routes of administration may incorporate intravenous, intradermal subcutaneous, intramuscular, intrathecal, epidural or intraperitoneal routes of injection. Known procedures, such as those conventionally employed by the pharmaceutical industry (e.g., in vivo experimentation, clinical trials, etc.), may be used to form specific formulations of the composition, the pharmaceutical composition or vaccine or therapeutic RNA molecule according to the invention and precise therapeutic regimes (such as doses of the agents and the frequency of administration). A “subject” may be a vertebrate, mammal, or domestic animal. Hence, compositions and medicaments according to the invention may be used to treat any mammal, for example livestock (e.g., a horse), pets, or may be used in other veterinary applications. Most preferably, however, the subject is a human being.
A “therapeutically effective amount” of the pharmaceutical composition or the vaccine or therapeutic RNA molecule is any amount which, when administered to a subject, is the amount of the aforementioned that is needed to produce a therapeutic effect. For example, a therapeutically effective amount of the composition, the pharmaceutical composition and the vaccine or therapeutic RNA molecule of the invention may comprise from about 0.0001 mg to about 800 mg of the payload molecule, preferably 0.001 mg to about 650 mg, and preferably from about 0.01 mg to about 500 mg of the payload molecule. A “pharmaceutically acceptable vehicle” as referred to herein, is any known compound or combination of known compounds that are known to those skilled in the art to be useful in formulating pharmaceutical compositions. In one embodiment, the pharmaceutically acceptable vehicle may be a solid, and the composition may be in the form of a powder or tablet. A solid pharmaceutically acceptable vehicle may include one or more substances which may also act as flavouring agents, lubricants, solubilisers, suspending agents, dyes, fillers, glidants, compression aids, inert binders, sweeteners, preservatives, dyes, coatings, or tablet- disintegrating agents. The vehicle may also be an encapsulating material. In powders, the vehicle is a finely divided solid that is in admixture with the finely divided active agents according to the invention. In tablets, the active agent (e.g., composition of the invention) may be mixed with a vehicle having the necessary compression properties in suitable proportions and compacted in the shape and size desired. The pharmaceutical vehicle may be a gel and the composition may be in the form of a cream or the like. However, the pharmaceutical vehicle may be a liquid, and the pharmaceutical composition is in the form of a solution. Liquid vehicles are used in preparing solutions, suspensions, emulsions, syrups, elixirs and pressurized compositions. The composition according to the invention may be dissolved or suspended in a pharmaceutically acceptable liquid vehicle such as water, an organic solvent, a mixture of both or pharmaceutically acceptable oils or fats. The liquid vehicle can contain other suitable pharmaceutical additives such as solubilisers, emulsifiers, buffers, preservatives, sweeteners, flavouring agents, suspending agents, thickening agents, colours, viscosity regulators, stabilizers or osmo-regulators. Suitable examples of liquid vehicles for oral
and parenteral administration include (partially containing additives as above, e.g., cellulose derivatives, preferably sodium carboxymethyl cellulose solution), alcohols (including monohydric alcohols and polyhydric alcohols, e.g., glycols) and their derivatives, and oils (e.g., fractionated coconut oil and arachis oil). For parenteral administration, the vehicle can also be an oily ester such as ethyl oleate and isopropyl myristate. Sterile liquid vehicles are useful in sterile liquid form compositions for parenteral administration. The liquid vehicle for pressurized compositions can be a halogenated hydrocarbon or other pharmaceutically acceptable propellant. Liquid pharmaceutical compositions, which are sterile solutions or suspensions, can be utilized by, for example, intramuscular, intrathecal, epidural, intraperitoneal, intravenous and subcutaneous injection. The composition of the invention may be prepared as any appropriate sterile injectable medium. The composition and/or the pharmaceutical composition of the invention may be administered orally in the form of a sterile solution or suspension containing other solutes or suspending agents (for example, enough saline or glucose to make the solution isotonic), bile salts, acacia, gelatin, sorbitan monoleate, polysorbate 80 (oleate esters of sorbitol and its anhydrides copolymerized with ethylene oxide) and the like. The composition of the invention and/or the pharmaceutical composition according to the invention can also be administered orally either in liquid or solid composition form. Compositions suitable for oral administration include solid forms, such as pills, capsules, granules, tablets, and powders, and liquid forms, such as solutions, syrups, elixirs, and suspensions. Forms useful for parenteral administration include sterile solutions, emulsions, and suspensions. All of the features described herein (including any accompanying claims, abstract and drawings), and/or all of the steps of any method or process so disclosed, may be combined with any of the above aspects in any combination, except combinations where at least some of such features and/or steps are mutually exclusive. For a better understanding of the invention, and to show how embodiments of the same may be carried into effect, reference will now be made, by way of example, to the accompanying Figures, in which:-
Figure 1A shows structures of various of locked nucleotides (LNs) or locked nucleic acids (LNA)/bridged nucleic acids (BNA) modifications. (A) LNA/BNA, (B) α-ʟ-LNA, (C) C2'-amino-LNA, (D) 3'-amino-2',4'-LNA, (E) seleno-LNA, (F) thio- LNA, (G) carba-LNA, (H) S-constrained ethyl (cEt) nucleic acid, (I) 2'-N-guanidino,4'- C-ethylene nucleic acid (GENA), (J) sulfonamide-bridged nucleic acid (suNA), (K) 2'- Me-LNA, (L) 6'-S-Me-2'-O,4'-C-ethylene-bridged nucleic acid (6'-S-Me-ENA), (M) triazole linked LNA. Figure 1B shows structures of various embodiments of locked nucleic acid nucleotide triphosphate (LNA-NTP): (A) LNA-ATP, (B) LNA-CTP, (C) LNA-5meCTP, (D) LNA- GTP, (E) LNA-UTP, and (F) LNA-TTP. Figure 2a shows that the efficient incorporation of locked NTPs only occurs at magnesium levels >20nm when using magnesium acetate. A) RNA yield ng/ul, B) RNA product as assessed by gel-electrophoresis. Figure 2b shows that the efficient incorporation of locked NTPs only occurs at magnesium levels >20nm when using magnesium chloride. A) RNA yield ng/ul, B) RNA product as assessed by gel-electrophoresis. Figure 3 illustrates the impact of individual LNA-NTP (ATP, CTP, GTP, UTP) substitution on mRNA expression of fLuc in HEK293T cells. mRNA encoding fLuc was generated by IVT, where individual nucleotides were substituted with 25, 75 or 90% LNA modified versions. HEK293T cells were transfected with 100ng of mRNA formulated in lipofectamine. A). Luciferase expression was monitored over 48h by measurement of relative light units (RLU, see methods). B) normalized RLU relative to unmodified mRNA. Figure 4 illustrates the impact of individual LNA-NTP (CTP, 5meCTP, UTP, TTP) substitution on mRNA expression of fLuc in HEK293T cells. mRNA encoding fLuc was generated by IVT, where individual nucleotides were substituted with 25, 75 or 90% LNA modified versions. HEK293T cells were transfected with 100ng of mRNA formulated in lipofectamine. A). Luciferase expression was monitored over 72h by measurement of relative light units (RLU, see methods). B) normalized RLU relative to unmodified mRNA.
Figure 5 illustrates the impact of LNA-NTP (ATP, CTP, GTP, UTP) substitution on mRNA expression of fLuc in HeLa cells. mRNA encoding fLuc was generated by IVT, where individual nucleotides were substituted with 25, 75 or 90% LNA modified versions. HeLa cells were transfected with 100ng of mRNA formulated in lipofectamine. A). Luciferase expression was monitored over 48h by measurement of relative light units (RLU, see methods). B) normalized RLU relative to unmodified mRNA. Figure 6 illustrates the impact of individual LNA-NTP (CTP, 5meCTP, UTP, TTP) substitution on mRNA expression of fLuc in HeLa cells. mRNA encoding fLuc was generated by IVT, where individual nucleotides were substituted with 25, 75 or 90% LNA modified versions. HeLa cells were transfected with 100ng of mRNA formulated in lipofectamine. A). Luciferase expression was monitored over 72h by measurement of relative light units (RLU, see methods). B) normalized RLU relative to unmodified mRNA. Figure 7 illustrates the impact of individual LNA-NTP (ATP, CTP, GTP, UTP) substitution on mRNA expression of fLuc in THP-1 cells. mRNA encoding fLuc was generated by IVT, where individual nucleotides were substituted with 25, 75 or 90% LNA modified versions. THP-1 cells were transfected with 100ng of mRNA formulated in lipofectamine. A). Luciferase expression was monitored over 48h by measurement of relative light units (RLU, see methods). B) normalized RLU relative to unmodified mRNA. Figure 8 illustrates the impact of individual LNA-NTP (CTP, 5meCTP, UTP, TTP) substitution on mRNA expression of fLuc in THP-1 cells. mRNA encoding fLuc was generated by IVT, where individual nucleotides were substituted with 25, 75 or 90% LNA modified versions. THP-1 cells were transfected with 100ng of mRNA formulated in lipofectamine. A). Luciferase expression was monitored over 72h by measurement of relative light units (RLU, see methods). B) normalized RLU relative to unmodified mRNA. Figure 9 illustrates the impact of individual LNA-NTP (ATP, CTP, GTP, UTP) substitution on the inflammatory profile of mRNA in THP-1 cells. Capped mRNA encoding firefly luciferase was generated by IVT, using wildtype (WT) NTPs, and where WT NTPs were substituted with 90% LNA-ATP, LNA-CTP, LNA-GTP or LNA-UTP.
Unmodified and modified mRNA were in 96-well plates of THP1 cells at 100ng in quadruplicate (Error bars represent n=4). At 48h post transfection, supernatants from each condition were then used to run an MSD assay to determine the different levels of cytokines stimulated by each RNA construct. Figure 10 illustrates the impact of different combinations LNA-NTPs (ATP, 5meCTP, GTP, TTP) substitution on mRNA expression of fLuc in HEK293T cells. mRNA encoding fLuc was generated by IVT, where individual nucleotides were substituted with combinations LNA NTPs. Where indicated, LNA-ATP, LNA-5meCTP and LNA- TTP were used at a 90% substitution, LNA-GTP at 75%. HEK293T cells were transfected with 25ng or 100ng of mRNA formulated in lipofectamine. A). Luciferase expression was monitored over 72h by measurement of relative light units (RLU, see methods). B) normalized RLU relative to unmodified mRNA. Figure 11 illustrates the impact of different combinations LNA-NTPs (ATP, 5meCTP, GTP, TTP) substitution on mRNA expression of fLuc in HeLa cells. mRNA encoding fLuc was generated by IVT, where individual nucleotides were substituted with combinations LNA NTPs. Where indicated, LNA-ATP, LNA-5meCTP and LNA-TTP were used at a 90% substitution, LNA-GTP at 75%. HeLa cells were transfected with 25ng or 100ng of mRNA formulated in lipofectamine. A). Luciferase expression was monitored over 72h by measurement of relative light units (RLU, see methods). B) normalized RLU relative to unmodified mRNA. Figure 12 illustrates the impact of different combinations LNA-NTPs (ATP, 5meCTP, GTP, TTP) substitution on mRNA expression of fLuc in THP1 cells. mRNA encoding fLuc was generated by IVT, where individual nucleotides were substituted with combinations LNA NTPs. Where indicated, LNA-ATP, LNA-5meCTP and LNA-TTP were used at a 90% substitution, LNA-GTP at 75%. THP-1 cells were transfected with 25ng or 100ng of mRNA formulated in lipofectamine. A). Luciferase expression was monitored over 72h by measurement of relative light units (RLU, see methods). B) normalized RLU relative to unmodified mRNA. Figure 13 illustrates the impact of combinations of LNA-NTP (LNA-ATP, LNA- 5meCTP, LNA-GTP, LNA-TTP) substitution on the inflammatory profile of mRNA in THP-1 cells. Capped mRNA encoding firefly luciferase was generated by IVT, using wildtype (WT) NTPs, and where WT NTPs were substituted with 90% LNA-ATP, LNA-
5meCTP, LNA-TTP and 75% LNA-GTP. and modified mRNA were transfected in 96-well plates of THP1 cells at 100ng in quadruplicate (Error bars represent n=3). At 48h post transfection, supernatants from each condition were then used to run an MSD assay to determine the different levels of cytokines stimulated by each RNA construct. Figure 14 illustrates the impact of different LNA-NTPs (90% LNA-ATP, LNA-CTP, LNA-TTP) substitution on saRNA expression of fLuc in HEK293T cells. saRNA encoding fLuc was generated by IVT, where nucleotides (ATP, CTP, TTP) were substituted with LNA NTPs. Where indicated, LNA-ATP, LNA-5meCTP and LNA-TTP were used at a 90% substitution. HEK293T cells were transfected with 25ng or 100ng of saRNA formulated in lipofectamine. A). Luciferase expression was monitored over 72h by measurement of relative light units (RLU, see methods). B) normalized RLU relative to unmodified saRNA. Figure 15 illustrates the impact of LNA-NTPs (90% LNA-ATP, LNA-CTP, LNA-TTP) substitution on saRNA expression of fLuc in HeLa cells. saRNA encoding fLuc was generated by IVT, where nucleotides (ATP, CTP, TTP) were substituted with LNA NTPs. Where indicated, LNA-ATP, LNA-5meCTP and LNA-TTP were used at a 90% substitution. HeLa cells were transfected with 25ng or 100ng of saRNA formulated in lipofectamine. A). Luciferase expression was monitored over 72h by measurement of relative light units (RLU, see methods). B) normalized RLU relative to unmodified saRNA. Figure 16 illustrates the impact of LNA-NTPs (90% LNA-ATP, LNA-CTP, LNA-TTP) substitution on saRNA expression of fLuc in THP-1 cells. saRNA encoding fLuc was generated by IVT, where nucleotides (ATP, CTP, TTP) were substituted with LNA NTPs. Where indicated, LNA-ATP, LNA-5meCTP and LNA-TTP were used at a 90% substitution. THP-1 cells were transfected with 25ng or 100ng of saRNA formulated in lipofectamine. A). Luciferase expression was monitored over 72h by measurement of relative light units (RLU, see methods). B) normalized RLU relative to unmodified mRNA. Figure 17 (a-j) shows similar data as shown in Figure 13, and illustrates cytokine concentrations in THP-1 supernatants after 24h of transfection with 100 ng of mRNA
with multiple LNAs. Bars represent (k) Heatmap of normalised concentration for each analyte. n=3. Figure 18 illustrates the impact of individual LNA-NTP (ATP, CTP, GTP, UTP) substitution on saRNA expression of fLuc expression at 24, 48 and 72h in a) HEK293T.17, b) HeLa and c) THP-1 cells transfected with 100 ng of single-modified LNA saRNA. saRNA encoding fLuc was generated by IVT, where individual nucleotides were substituted with 25, 75 or 90% LNA modified versions. d-f) RLU fold-change relative to unmodified at each timepoint. Bars represent mean ± SEM, n=3. Figure 19 (a-j) illustrates cytokine concentrations in THP-1 supernatants after 24h of transfection with 100 ng of single-modified LNA saRNA. Bars represent mean ± SEM. (k) Heatmap of normalised concentration for each analyte. n=3. Figure 20 is a schematic chart for the 10 conditions trialled with different modification rates for saRNA. LNA-ATP, -CTP and -UTP tested at 90% molar substitution; LNA-GTP tested at 75% Figure 21 illustrates the impact of different combinations LNA-NTPs (ATP, CTP, GTP, TTP) substitution on saRNA expression of fLuc at 24, 48 and 72h in a) HEK293T.17, b) HeLa and c) THP-1 cells transfected with 100 ng of saRNA with multiple LNAs. d-f) RLU fold-change relative to unmodified at each individual timepoint. Bars represent mean ± SEM, n=3. Figure 22 (a-j) illustratesd cytokine concentrations in THP-1 supernatants after 24h of transfection with 100 ng of saRNA with multiple LNAs. Bars represent mean ± SEM. (k) Heatmap of normalised concentration for each analyte. n=3. Figure 23 illustrates luciferase expression in human skin explants injected intradermally with 2.5 μg of RNA inLNPs. a) Quantification of total emission expressed as photons/sec of skin explants injected with saRNA constructs. b) Quantification of total emission expressed as photons/sec of skin explants injected with mRNA constructs c) Area under the curve of total emission of all samples. Significance calculated by ordinary one-way ANOVA test corrected with Tukey’s multiple comparisons test (p-value < 0.05 *, <0.01 **, 0.001 ***). d) Image of explant layout pre-quantification 72h post injection. Mean ± SEM for three technical replicates of n=2.
Figure 24 illustrates luciferase expression in BALB/C mice receiving two intramuscular injections with 2.5 μg of LNP formulated RNA. a) Quantification of mice's total emission (photons/sec) at days 1, 3, 7, 14, 21, 31, and 45. b) Quantification of total emission (photons/sec) by days to day 21. Significance calculated by two-way ANOVA test corrected with Tukey’s multiple comparisons test (p-value < 0.05 *, <0.01 **, <0.001 ***, <0.0001 ****). c) Area under the curve (AUC) of total emission for 21 days with ordinary one-way ANOVA significance test corrected with Tukey’s multiple comparisons test. d) % Drop in baseline weight for 7 days post-injection. Mean for two technical replicates of n=5, statistical comparisons performed across all samples within the same timepoint. Figure 25 (a-j) illustrates cytokine concentrations in BALB/C mice serum at baseline and 4h post intramuscular injections of 5 μg of RNA in C12-200. Mean with individual values, Two-way ANOVA test corrected with Tukey’s multiple comparisons test statistical comparisons performed across all samples within the same timepoint (p- value < 0.05 *, <0.01 **, <0.001 ***, <0.0001 ****). (k) Heatmap of normalised concentration for each analyte at 4hpi. n=5. Examples The inventors investigated the effects of the incorporation of locked nucleic acids (LNA) into RNA sequences (mRNA and saRNA) of greater than 25 nucleotides (NTPs), by in vitro transcription. It is demonstrated for the first time that incorporation of LNA- NTPs both increases the level and duration of protein expression in transduced cells, and that mRNA containing LNA-NTPs is more stable, and can be translated by cellular ribosomes to generate functional proteins. Materials and Methods In vitro transcription (IVT) mRNA encoding fLuc was produced by in-vitro transcription (IVT) using a linearised DNA template. The final volume for each IVT reaction was 50uL, and the standardised IVT reaction mix was as follows: Table 1 - Standardised IVT reaction mix 0.5 μg DNA template 40mM DTT
40mM HEPES 4U RNase Inhibitor per 1 μg DNA 100U T7 RNA polymerase per 1 μg DNA 75mM Mg(OAc)2 10mM Na(OAc) 75mM Mg(OAc) 10mM of each GTP, ATP, CTP, UTP 0.2mM Spermidine The total concentration of each NTP was kept at 10nM, however, the relative proportion of unmodified to modified NTP was varied as indicated. For example, where it is described that 75% GTP was substituted with LNA-GTP, it indicates that the reaction contained 7.5mM LNA-GTP plus 2.5mM GTP. IVT reactions were performed at 370C for 4 h. RNA yield was then measured right after IVT using the Qubit RNA Broad Range Assay kit with the Qubit Fluorometer (Thermo Fisher, UK) according to the manufacturer’s protocol. Prior to post capping, RNA was purified using Lithium Chloride precipitation. Post capping of RNA was performed using ScriptCapTM Cap 1 Capping System (CellScript, UK) and reaction was incubated at 370C for 2h. After post capping, RNA was purified again using lithium chloride precipitation and the final RNA concentration was measured using Nanodrop One (Thermo Scientific, UK). To assess the quality of the RNA, purified RNAs and the RNA Millennium Marker Ladder (Thermo Fisher, UK) were mixed with 2x NorthernMax-Gly Sample Loading Dye (Thermo Fisher, UK) and incubated at 50 °C for 30 min to denature the RNA. A 1.2 % agarose gel with 1x NorthernMax Running Buffer (Thermo Fisher, UK) was prepared. After incubation, the denatured ladder and samples were loaded on to the gel and the gel was run at 80 V for 45 min. The gel was then imaged on a GelDoc-It2 (UVP, UK). Cell culture and in vitro transfection HEK293T.17 (ATCC, UK) and HELA (ATCC) cells were cultured in complete Dulbecco’s Modified Eagle’s Medium (DMEM) (Gibco, UK) containing 10% fetal bovine serum (FBS), 1% L-glutamine (L-glu) and 1% penicillin-streptomycin (Pen-strep) (Thermo Fisher Scientific, UK). THP1 cells (ATCC) were cultured in complete Roswell Park Memorial Institute (RPMI) 1640 medium with 10% FBS, 1% L-glu and 1% pen-strep. Cells were plated in a 96-well plate 24 h prior to transfection at a density of 7 x10
4 cells
per well for HEK293T.17 and HeLa 1x10
5 cells per well THP-1 cells. Transfection of saRNA and mRNA encoding fLuc was performed using Lipofectamine MessengerMAX (Thermo Fisher, UK) according to the manufacturer’s instructions. In Vitro Firefly luciferase assay At 24, 48 or 72 h post transfection, half of the cell supernatants were discarded from each well and an equal volume of the Bright-GloTM Luciferase (Promega, UK) added to each well then placed in the dark. Cells were incubated with the luciferase reagent for 5 min to ensure complete lysis of the cells. Cells were then pipetted up and down and transferred to a white 96-well Costar® plate (Thermo Fisher Scientific). The luminescence was then measured on the FLUOstar Omega microplate reader (BMG Labtech) using gain 2500, 3000, 3600 and 4095. In Vitro Cytokine Profiling Cell supernatants were collected at 24 and 48 h post transfection with saRNA or mRNA and cytokines measured using the U-PLEX Biomarker Group 1 (Human) Multiplex Assay (Meso Scale Discovery, US) according to the manufacturer’s instructions. Plates were kindly coated with Linker-coupled capture antibodies and were stored in 4°C for no more than 7 days until further analysis. The cytokine panel selected for this study includes GRO- ^, IFN- ^2a, IFN- ^, IFN- ^, IP-10, MCP-1, MCP-2, MCP-3, MIP-1 ^, MIP- 1 ^. Plates were read on a MESO QuickPlex SQ 120MM (Meso Scale Discovery) and data were analyzed with MSD Discovery Work bench software (Meso Scale Discovery). Lipid nanoparticle (LNP) formulation of RNA RNA was encapsulated in LNPs consisting of C12-200, DSPC, cholesterol and DMPE- PEG2000 at molar % of 35, 16, 46.5, and 2.5, respectively. Using a NanoAssemblr® Ignite Microfluidics system (Precision Nanosystems, Canada), the RNA in the acidic solution and the lipid mix diluted in ethanol were self-assembled at a 3:1 RNA to Lipid ratio, N/P ratio of 8 and flow rate of 8 ml/min. The particles were diluted to 5X the volume in PBS+10% sucrose, ethanol was removed, and particles were concentrated using 10kDa Amicon Ultr-15 tubes (Sigma-Aldrich, US) following manufacturer’s guidelines. The encapsulated RNA's final concentration and efficiency were measured using a Ribogreen Assay (Life Technologies, US) following the manufacturer’s guidelines. The particle size and polydispersity index (PDI) were measured by dynamic light scattering (DLS) using a Zetasizer Nano ZS (Malvern Instruments Ltd, UK), and
the instrument was also used to surface charge of the particles. LNP formulations were deemed successful if encapsulation was >80%, particle size ranged from 80-120 nm, PDI was <0.2, and Z-potential was between -2 to -6 mV. LNPs were stored at -80ºC until use. Injection, Culture, and Imaging of Human Skin Explants Remains of surgically removed human skin samples from patients undergoing mastectomy surgeries were obtained after receiving signed informed consent from all patients under protocols approved by the Local Research Ethics Committee. Tissue was freshly collected from the hospital post-surgery and transferred to the laboratory for immediate processing. The subcutaneous fat layer was carefully removed, the epidermis cut into 1cm
2 bits, and transferred into 24-well plates containing 1ml of DMEM supplemented with 10% FBS, 1% l-glutamine and 1% penicillin/streptomycin. Daily media changes were performed for 22 days, and samples discarded appropriately. One day after sample processing, explants were injected intradermally with 2.5 μg of formulated RNA in 50 μl of PBS+10% sucrose with a Micro-Fine Demi 0.3 mL syringe (Becton Dickinson, UK). On days 1, 3, 5, 7, 10, 14 and 21 post-injections, media was supplemented to a final concentration of 3 mg/mL and imaged after 10 minutes in the AMI HTX (Spectral ImagingSystems, US), and total emission (photons/sec) quantified using the Aura Imaging Software (Spectral ImagingSystems, US). Mouse injections, imaging, and cytokine analysis BALB/c female mice aged 6–8 weeks old were placed into groups of n=5. Animals were handled, and procedures were performed under the terms of a project license granted under the UK Home Office Animals Act 1986. All the procedures and protocols used in this study were approved by an animal ethical committee, the Animal Welfare and Ethical Review Body (AWERB). In each hind leg, mice received an intramuscular injection (i.m., quadriceps) with 2.5 ug of formulated RNA in 50 μl. Mice were bled by the tail vein at baseline, and 4h post-injection, and serum was collected by centrifugation at 10000 rpm for 10 min at 4ºC for cytokine analysis. On days 1, 3, 7, 14, 21, 31 and 45 mice received 100 μl intraperitoneal (i.p.) injections of 30 ug/mL XenoLight RediJect D-Luciferin (PerkinElmer, UK), were anaesthetised under a concentration of 3% Isofluorane (Dechra, UK) in oxygen, imaged in the AMI HTX, and total emission (photons/sec) was quantified using the Aura Imaging Software. Cytokine expression from collected mice sera were analysed using a Meso Scale Discovery (MSD) mouse 10-spot U-PLEX kit (K15069M-2) for the following analytes: KC, IFN-γ, IL-6,
IL-12p70, IP-10, MCP-1, MIP-1α, MIP- α, and IFN-β. Samples were prepared following manufacturer’s guidelines, the plate was read using the Methodical Mind Software (MSD, US), and data was analysed using the MSD Discovery Workbench Software (MSD, US). Example 1 – The incorporation of locked-NTPs (LNA-NTP) can be achieved when using magnesium ion concentrations of greater than 20mM The inventors have surprisingly demonstrated that incorporation of ribose modified LNA-NTP can be achieved when using magnesium ion concentrations that are greater than 20mM (see Figure 2a). In particular, the inventors have observed that magnesium acetate concentrations of greater than 20mM are required for efficient incorporation of LNA-GTPs, and ideally concentrations of ≤75mM. However, the inventors believe that the maximum concentration of magnesium acetate could even be greater than 80mM. Alternatively, the inventors also observed that magnesium chloride concentrations of greater than 20mM can be used for efficient incorporation of LNA-GTPs, and ideally concentrations of 40mM (Figure 2b), although RNA yields were occasionally less optimal than with magnesium acetate. All experiments were performed with wild type T7 polymerase, however, the inventors expect that the reaction conditions described herein would also be advantageous for mutated versions to T7 polymerase shown to be more permissive for NTP substitutions, and also other RNA polymerases including T7, T3 and SP6, and potential mutated variants. As described in the following examples, the inventors then assessed the impact of incorporation of individual LNA-NTPs on the function of mRNA in three cell lines: Human embryonic kidney 293T (HEK293T) cells that have impaired innate sensing mechanisms that may lead to suppressed RNA expression (Example 2), HeLa cells representative of epithelial cells (Example 3), and THP-1 cells representative of myeloid cells (Example 4), the latter two displaying robust innate responses to naked unmodified RNA. Example 2 – Incorporation of individual LNA-NTPs increases both the magnitude and expression of capped mRNA in cells that have impaired innate sensing mechanisms for recognizing RNA (e.g. HEK293T cells)
The mRNA construct used in these encodes the sequence for Firefly luciferase (fLuc) and expression of luciferase is detected by luciferase activity in cells overtime as an indicator of the translation of a functional protein. In transfection experiments performed in HEK293T cells when using 100ug of mRNA formulated with lipofectamine, the inventors observed that incorporation of LNA-ATP, LNA-CTP, each increased luciferase expression over 48hs (Figure 4) with a fold increase of ranging from 2-fold with 25% substitutions to 4-fold with 90% substitution (Figure 3). Surprisingly, substitution of LNA-GTP had a greater impact on expression with 25% substitution than with 90% substitution. Further experiments were conducted to assess the impact of LNA-5meCTP, often incorporated into modified oligonucleotides and TTP representative of a DNA NTP (Figure 4). Data from these experiments that CTP and 5meCTP had similar properties with respect to enhancing the expression of mRNA. Likewise, there was little or no difference between UTP and TTP with respect to improved expression of fLuc. These data indicate that incorporation of individual LNA- NTPs increases both the magnitude and expression of uncapped mRNA in cells that have impaired innate sensing mechanisms for recognizing RNA. This suggests that mRNA modified with individual LNA-NTPs may provide enhanced expression and stability even in cells with impaired RNA sensing mechanisms. These data indicate that incorporation LNA-NTPs and in particular LNA-UTP, LNA- TTP, LNA-CTP, LNA-5meCTP, LNA-ATP and LNA-GTP enhances the magnitude and duration of mRNA expression in cells that have impaired innate RNA sensing that may suppress RNA translation and increase degradation. The use of LNA-5meCTP exemplifies the use of base modified LNA-NTPs. The inventors anticipate that further gains will be realized when individual LNA-NTPs are used in combination: For example, for mRNA, suitable combinations are: 1.75% LNA-ATP+90%LNA-5meCTP 2.90% LNA-ATP+90%LNA-CTP 3.90% LNA-ATP+75% LNA-GTP 4.90% LNA-ATP+90% LNA-TTP 5.90% LNA-ATP+90% LNA-UTP 6.90% LNA-5meCTP+75% LNA-GTP 7.90% LNA-CTP+75% LNA-GTP 8.90% LNA-5meCTP+90% LNA-TTP 9.90% LNA-CTP+90% LNA-TTP 10.90% LNA-5meCTP+90% LNA-UTP
11.90% LNA-CTP+90% LNA-UTP 12.75% LNA-GTP+90% LNA-TTP 13.75% LNA-GTP+90% LNA-UTP 14.90% LNA-ATP+90% LNA-5meCTP+75%LNA-GTP 15.90% LNA-ATP+90% LNA-CTP+75%LNA-GTP 16.75% LNA-ATP+90% LNA-5meCTP+90% LNA-TTP 17.75% LNA-ATP+90% LNA-CTP+90% LNA-TTP 18.75% LNA-ATP+90% LNA-5meCTP+90% LNA-UTP 19.75% LNA-ATP+90% LNA-CTP+90% LNA-UTP 20.75% LNA-ATP+75% LNA-GTP+90% LNA-TTP 2175% LNA-ATP+75% LNA-GTP+90% LNA-UTP 22.90% LNA-5meCTP+75% LNA-GTP+90% LNA-TTP 23.90% LNA-CTP+75% LNA-GTP+90% LNA-TTP 24.90% LNA-5meCTP+75% LNA-GTP+90% LNA-UTP 25.90% LNA-CTP+75% LNA-GTP+90% LNA-UTP 26.75% LNA-ATP+90% LNA-5meCTP+75% LNA-GTP+90% LNA-TTP 27.75% LNA-ATP+90% LNA-CTP+75% LNA-GTP+90% LNA-TTP 28.75% LNA-ATP+90% LNA-5meCTP+75% LNA-GTP+90% LNA-UTP 29.75% LNA-ATP+90% LNA-CTP+75% LNA-GTP+90% LNA-UTP, or any other percentage ratios. Example 3 – Incorporation of individual LNA-NTPs increases the magnitude and expression of capped mRNA in cells that have intact innate sensing mechanisms for recognizing RNA (e.g. HeLa cells) Next, the inventors assessed the impact of incorporation of individual LNA-NTPs (ATP, CTP, GTP, UTP) on the function of mRNA in HeLa cells representative of epithelial cells. Unlike the HEK293T cells tested in the previous example, HeLa cells have intact epithelial innate sensing mechanisms able to respond to RNA triggering intracellular signaling pathways able to reduce RNA expression and enhance the rate of RNA degradation [3-5, 12, 13]. Substitution of individual NTPs with locked NTPs enhances fLuc expression in HeLa epithelial cells by up to 10-fold at 24 hours (LNA-UTP) and 60-fold (LNA-UTP) at 48h. Detectable changes were still observed for substitutions each of the individual substitutions. These data indicate that the incorporation of LNA-NTPs enhance both the magnitude and duration of expression in epithelial cells with intact innate sensing
pathways (Figure 5 & 6), where the on expression for the individual NTPs is UTP=TTP>CTP=5meCTP>ATP>GTP. It is anticipated that the use of different LNA-NTPs in combinations will lead to further gains in expression. Example 4 – Incorporation of individual LNA-NTPs increases the magnitude and expression of capped mRNA in cells that have intact innate sensing mechanisms for recognizing RNA (e.g. THP-1 cells) Next, the inventors assessed the impact of incorporation of individual LNA-NTPs (ATP, CTP, GTP, UTP) on the function of mRNA in THP-1 cells representative of monocytic cells representative of myeloid cells, specifically monocytic cells. These cells are particularly resistant to transfection due to sensitive innate sensing mechanisms able to respond to RNA triggering intracellular signaling pathways able to reduce RNA expression and enhance the rate of RNA degradation [3-5, 12, 13]. Substitution of individual NTPs with locked NTPs enhances fLuc expression in THP-1 monocytic cells by up to 10-fold at 24 hours (LNA-UTP) and 20-fold (LNA-UTP) at 48h (Figure 7 & 8). Detectable changes were still observed for substitutions each of the individual substitutions (LNA-ATP, -CTP, -5meCTP, -GTP, -TTP, UTP (Figures 5 & 6). These data indicate that the incorporation of LNA-NTPs enhance both the magnitude and duration of expression in monocytic cells with intact innate sensing pathways (Figures 5 & 6), where the impact hierarchy on expression for the individual NTPs is UTP=TTP>CTP=5meCTP>ATP>GTP. It is anticipated that the use of different LNA- NTPs in combinations will lead to further gains in expression. Example 5 – Summary of the data Table 2 below summarises the impact of LNA modifications on mRNA expression. Table 2 - The impact of locked modifications on mRNA expression when using 100ng mRNA with 90% substitution modification HEK293T HeLa THP-1 LNA-ATP + ++ + LNA-CTP + +++ + LNA-5meCTP + +++ + LNA-GTP +/- + + LNA-TTP + +++ +++ LNA-UTP + +++ +++ + = 1-10 fold, ++ = 10-19 fold, +++ = ≥ 20 fold
Example 6 - Incorporation of NTPs influences the induction of inflammatory cytokines indicative of innate responses to RNA in THP-1 cells. To determine whether substitution of individual LNA-NTPs could reduce the activation of innate sensing, interferon generation and/or degradation of mRNA in host cells, THP1 cells were transfected with unmodified vs. mRNA modified with individual LNPs and the cell supernatant harvested at 24 and 48 h post transfection to characterize the induced cytokine profile. THP1 cells were chosen for this analysis because they represent monocyte cells with very robust innate responses. A Meso Scale Discovery (MSD) assay was used to measure the cytokine levels where the cytokine panel selected for this experiment included: GRO-α, IFN- ^ IFN-β, IFN- ^, IP-10, MCP-1, MCP-2, MCP- 3, MIP-1α, and MIP-1β. It was observed that substitution of individual NTPs with LNA- NTPs was able to influence the secretion profile of a panel of cytokines in supernatants harvested from transfected THP-1 cells (Figure 9), in particular LNA-GTP was able to reduce IFN-alpha, LNA-GTP and UTP reduced IP-10 and all four LNA-NTPs (ATP, CTP, GTP, UTP) reduced MCP1 and MCP2 with a hierarchy of GTP>UTP>CTP>ATP. These data demonstrate that individual LNA-NTP have potential to impact on innate recognition and associated cytokine induction. Example 7 - Combined LNA-NTP substitutions increase both the magnitude and expression of capped mRNA in cells that have impaired innate sensing mechanisms for recognizing RNA (e.g. HEK293T cells) Having determined that substitution of individual LNA-NTPs enhanced fLuc expression, the inventors determined whether further gains in expression could be delivered by combined LNA-NTP substitutions. FLuc mRNA was generated by IVT: using unmodified NTPs; and where nucleotides were substituted with ≥ 75% LNA-GTP, ≥ 90% LNA- ATP, 90% LNA-5meCTP or 90% LNA-TTP alone, or in different combinations. These constructs were used to transfect HEK293T cells, and Luciferase expression was monitored over 72h by measurement of relative light units. Maximal enhancement (up to 12-fold at 24h) was seen with combined substitution of LNA- ATP+LNA-CTP+LNP-TTP at a 100ng dose (Figure 10). A similar level of enhancement was seen with substitutions of all four NTPs. These data suggest that combined LNA- NTPs have a greater potential to enhance expression in the absence of innate recognition than individual LNA-NTP substitutions (Figure 3).
Example 8 - Combined LNA-NTP increase both the magnitude and expression of capped mRNA in cells that have intact innate sensing mechanisms for recognizing RNA (e.g. HeLa cells) Subsequently, the inventors assessed the impact of incorporation of LNA-NTP combinations on the function of mRNA in HeLa-1 cells representative of epithelial cells. These cells have intact innate sensing mechanisms able to respond to RNA triggering intracellular signaling pathways able to reduce RNA expression and enhance the rate of RNA degradation [3-5, 12, 13]. FLuc mRNA was generated by IVT: using unmodified NTPs; and where nucleotides were substituted with ≥ 75% LNA-GTP, ≥ 90% LNA- ATP, 90% LNA-5meCTP or 90% LNA-TTP alone or in different combinations. These constructs were used to transfect HeLa cells, and Luciferase expression was monitored over 72h by measurement of relative light units (Figure 11). Here combined substitution of LNA-ATP+LNA- CTP+LNA-TTP at a 100ng dose demonstrated >200-fold enhancement at 28h and 72h. A similar, but lower level of enhancement was seen with substitutions of all four NTPs. These data indicate that the incorporation of combined LNA-NTP substitutions has an even greater impact on both the magnitude and duration of expression in cells with intact innate sensing pathways, here demonstrated using an epithelial cell line. Example 9 - Combined LNA-NTP substitutions increase both the magnitude and expression of capped mRNA in cells that have intact innate sensing mechanisms for recognizing RNA (e.g. THP-1 cells) Next, the inventors assessed the impact of incorporation of LNA-NTP combinations on the function of mRNA in THP-1 cells representative of monocytic cells representative of myeloid cells, specifically monocytic cells. These cells are particularly resistant to transfection due to sensitive innate sensing mechanisms able to respond to RNA triggering intracellular signaling pathways able to reduce RNA expression and enhance the rate of RNA degradation [3-5, 12, 13]. FLuc mRNA was generated by IVT: using unmodified NTPs; and where nucleotides were substituted with ≥ 75% LNA-GTP, ≥ 90% LNA- ATP, 90% LNA-5meCTP or 90% LNA-TTP alone or in different combinations. These constructs were used to transfect THP1 cells, and Luciferase expression was monitored over 72h by measurement of relative light units (Figure 12). Here, combined substitution of LNA-ATP+LNA- CTP+LNA-TTP at a 100ng dose demonstrated 200-fold enhancement at 24h and up to
600-fold enhancement at 48h. A of enhancement was seen with substitutions of all four NTPs. These data indicate that the incorporation of combined LNA-NTP substitutions has an even great impact on both the magnitude and duration of expression in cells with intact innate sensing pathways, here demonstrated using a monocytic cell line. Example 10 – Summary of the data Table 3 below summarises the impact of LNA combinations on mRNA expression. Table 3 - The impact of LNA-modifications on mRNA expression when using 100ng mRNA where nucleotides were substituted with ≥ 75% LNA-GTP, ≥ 90% LNA- ATP, 90% LNA-5meCTP or 90% LNA-TTP alone or in different combinations. modification HEK293T HeLa THP-1
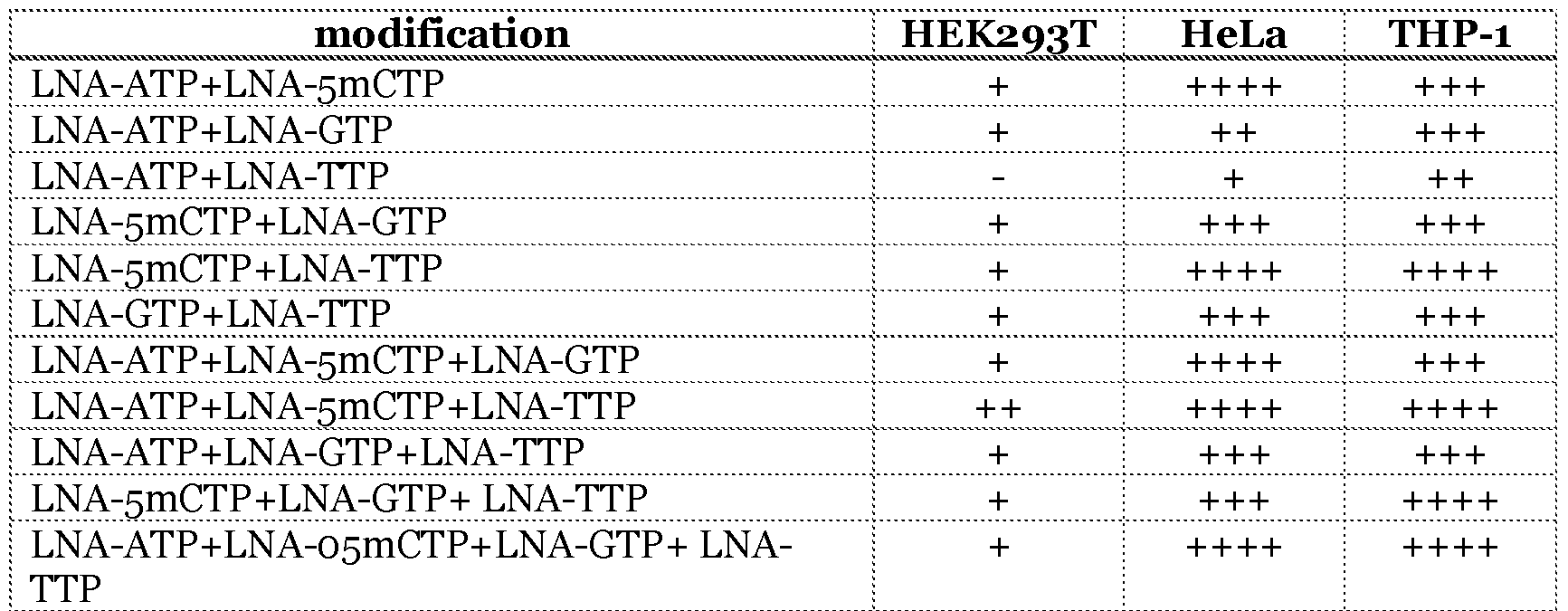
Example 11 - Incorporation of LNA-NTP combinations influences the induction of inflammatory cytokines indicative of innate responses to RNA in THP-1 cells. To determine whether substitution with combinations of LNA-NTPs could reduce the activation of innate sensing, interferon generation and/or degradation of mRNA in host cells, THP1 cells were transfected with RNA containing unmodified NTPs; and where nucleotides were substituted with ≥ 75% LNA-GTP, ≥ 90% LNA- ATP, 90% LNA- 5meCTP or 90% LNA-TTP alone or in different combinations. Cell supernatant harvested at 24 and 48 h post transfection to characterize the induced cytokine profile. THP1 cells were chosen for this analysis because they represent monocyte cells with very robust innate responses. A Meso Scale Discovery (MSD) assay was used to measure the cytokine levels where the cytokine panel selected for this
experiment included GRO-α, IFN- ^ ^, IP-10, MCP-1, MCP-2, MCP-3, MIP- 1α, MIP-1β. It was also observed that substitution of combined LNA-NTPs (Figure 13) had a greater impact than individual LNA-NTPs with respect to the secretion profile of an indicative panel of innate cytokines in supernatants harvested from transfected THP-1 cells (Figure 9). Here two, three and four LNA-NTP combinations were able to suppress MCP-1, MCP-2, MIP-1 alpha and MIP-1 beta, and reduce expression of Gro-alpha, IFN- beta, IFN-gamma, IP10 and MC3, where substitution with all four LNA-NTPs was the most effective at suppressing responses across all the analytes tested (Figure 13). Referring to Figure 17, there is shown alternative data for this experiment in which Figure 17 a-j show the cytokine concentrations in THP-1 supernatants after 24h of transfection with 100 ng of mRNA with multiple LNAs. Figure 17k is a heatmap of normalised concentration for each analyte. Example 12 - Combined LNA-NTP substitutions increase both the magnitude and expression of capped saRNA in cells that have impaired innate sensing mechanisms for recognizing RNA (e.g. HEK293T cells) Having that LNA-NTPs enhanced mRNA fLuc expression, the inventors determined whether the impact of LNPs could also contribute to the expression of fLuc when using self-amplifying RNA. This would test if the impact of LNA-NTPs were applicable to different types of encoding RNA. FLuc saRNA was generated by IVT: using unmodified NTPs; and where nucleotides were substituted with ≥ 90% LNA- ATP, 90% LNA- 5meCTP and 90% LNA-TTP. These constructs were used to transfect HEK293T cells, and Luciferase expression was monitored over 72h by measurement of relative light units. Maximal enhancement (up to 14-fold at 48h) was seen with combined substitution of LNA-ATP+LNA-CTP+LNP-TTP at a 25ng dose (Figure 14). These data indicate that the incorporation of combined LNA-NTP substitutions can also impact both the magnitude and duration of saRNA expression in HEK293T cells. Example 13 - Combined LNA-NTP substitutions increase both the magnitude and expression of capped saRNA in cells that have intact innate sensing mechanisms for recognizing RNA (e.g. HeLa cells) Subsequently, the inventors assessed the impact of incorporation of LNA-NTP combinations on the function of saRNA in HeLa-1 cells representative of epithelial
cells. These cells have intact innate able to respond to RNA triggering intracellular signaling pathways able to reduce RNA expression and enhance the rate of RNA degradation [3-5, 12, 13]. FLuc saRNA was generated by IVT: using unmodified NTPs; and where nucleotides were substituted with ≥ 90% LNA- ATP, 90% LNA-5meCTP or 90% LNA-TTP. These constructs were used to transfect HeLa cells, and Luciferase expression was monitored over 72h by measurement of relative light units (Figure 15). Here combined substitution of LNA-ATP+LNA-CTP+LNA-TTP at a 100ng dose demonstrated a 200- fold enhancement at 72h. A similar, but lower level of enhancement was seen with substitutions of all four NTPs. These data indicate that the incorporation of combined LNA-NTP substitutions can also impact both the magnitude and duration of saRNA expression in cells with intact innate sensing pathways, here demonstrated using an epithelial cell line. Example 14 - Combined LNA-NTPs substitution increase both the magnitude and expression of capped saRNA in cells that have intact innate sensing mechanisms for recognizing RNA (e.g. THP-1 cells) Next, the inventors assessed the impact of incorporation of LNA-NTP combinations on the function of saRNA in THP-1 cells representative of monocytic cells representative of myeloid cells, specifically monocytic cells. These cells are particularly resistant to transfection due to sensitive innate sensing mechanisms able to respond to RNA triggering intracellular signaling pathways able to reduce RNA expression and enhance the rate of RNA degradation [3-5, 12, 13]. FLuc saRNA was generated by IVT: using unmodified NTPs; and where nucleotides were substituted with ≥ 90% LNA- ATP, 90% LNA-5meCTP and 90% LNA-TTP. These constructs were used to transfect THP1 cells, and Luciferase expression was monitored over 72h by measurement of relative light units (Figure 16). Here combined substitution of LNA-ATP+LNA-CTP+LNA-TTP at a 100ng dose demonstrated 100-fold enhancement at 24h and up to 1000-fold enhancement at 72h. These data indicate that the incorporation of combined LNA-NTP substitutions can also impact on both the magnitude and duration of saRNA expression in cells with intact innate sensing pathways, here demonstrated using a monocytic cell line. Example 15 - Effect of single modifications of LNA-NTPs on saRNA expression in vitro
To determine whether saRNA modified individual LNA-NTPs enabled RNA transcription. RNA modified at ratios of 25, 75 and 90% LNA was synthesised, and RNA expression was assessed in HEK293T.17, HeLa and THP-1 cells after 24, 48 and 72h from transfection (Figure 18). Luciferase expression was obtained for all RNA conditions in HEK293T.17 cells, with a modest enhancement of expression for LNA- GTP compared to the unmodified sample. In HeLa cells, a 40-fold increase was observed for the LNA-GTP samples at 72h relative to the unmodified sample, and a 90- fold increase to the unmodified was obtained for the 90% LNA-UTP at the same time- point. THP-1 cells displayed significantly enhanced expression for 75% LNA-ATP, 75% LNA-GTP and 90% LNA-GTP after 72h, with 40, 70 and 175-fold increases, respectively. To determine whether saRNA modified with individual LNA-NTPs, induced cytokine expression was measured by MSD (Figure 19). Expression of GRO-α, IFN-α2a, IFN-β and MCPs were decreased relative to unmodified saRNA in the following order: LNA-CTP< LNA-UTP< LNA-ATP<LNA-GTP. All the LNA-NTPs significantly reduced MCP-1, while 90% LNA-GTP and –UTP led to a significantly lower expression of MIP-1α and MIP-1β. In contrast, no difference in innate signalling to the unmodified control was observed for IFN-γ and IP-10. Example 16 – Summary of the data Table 4 below summarises the impact of LNA modifications on mRNA expression. Table 4 - The impact of locked modifications on saRNA expression when using 100ng mRNA with 90% substitution modification HEK293T HeLa THP-1 LNA-ATP +++ +++ LNA-CTP + ++ LNA-GTP + +++ ++++ LNA-UTP +++ + + = 1-10 fold, ++ = 10-19 fold, +++ = ≥ 20 fold, ++++ = ≥ 100 fold Example 17 - Effect of combined modifications of LNA-NTPs on saRNA in vitro Having established the impact of the LNA on saRNA at an individual level the inventors assessed the impact of modifying multiple LNAs in across 10 different combinations (Figure 20). Expression levels of the combined modifications in saRNA were assessed
after 24, 48 and 72h following 21). There were modest changes in luciferase expression, ranging between a 5 and 10-fold increase in expression at 24h in HEK293T.17 cells, which dropped in the following days. In HeLa cells, maximal expression was seen at 24h with significant increases in expression for combinations (C) C1-C4 and C8-10. However, fold change peaked at 72h for all samples, with combinations (C) C3 (LNA-ATP+LNA-UTP), C8 (LNA-ATP+LNA-CTP+LNA-UTP), C9 (LNA-ATP+LNA-GTP+LNA-UTP) and C10 (LNA-CTP+LNA-GTP+ LNA-UTP) significantly increasing by 700, 2350, 1000 and 2000-fold, respectively, reflecting a longer duration of expression relative to the unmodified control. Transfection studies using THP-1 cells presented a highly similar trend, with maximal expression was seen at 24h with significant increases in expression for combinations (C) C1-C4 and C7-10. However, fold change peaked after 72h. Significance was observed for C1 (LNA- ATP+LNA-CTP), C3, C7 (LNA-ATP+LNA-CTP+LNA-GTP), C8, C9, and C10, with fold- changes of 235, 350, 335, 565, 400, and 550, respectively, also reflecting a longer duration of expression relative to the unmodified control. Cytokine analysis of the LNA combinations in saRNA revealed a mixed profile (Figure 22). LNAs did not affect IP-10 levels, mildly lowered GRO-α and MIP-1β expression, and had pronounced effects on the IFN-α2a, -β and -γ, the MCPs and MIP-1α. All conditions caused significantly decreased MCP-1 levels. Example 18 – Summary of the data Table 5 below summarises the impact of LNA combinations on saRNA expression. Table 5 - The impact of LNA-modifications on saRNA expression when using 100ng mRNA where nucleotides were substituted with 75% LNA-GTP, 90% LNA- ATP, 90% LNA-CTP and/or 90% LNA-UTP alone or in different combinations at 72h following transfection. Condition modification HEK293T HeLa THP-1 1 LNA-ATP+LNA-CTP + +++ +++ 2 LNA-ATP+LNA-GTP + +++ +++ 3 LNA-ATP+LNA-UTP + +++ +++ 4 LNA-CTP+LNA-GTP + +++ +++ 5 LNA-CTP+LNA-UTP + +++ +++ 6 LNA-GTP+LNA-UTP + +++ +++
7 LNA-ATP+LNA- + +++ +++ 8 LNA-ATP+LNA-CTP+LNA-UTP + ++++ +++ 9 LNA-ATP+LNA-GTP+LNA-UTP + ++++ +++ 10 LNA-CTP+LNA-GTP+ LNA-UTP + ++++ +++ + = 1-10 fold, ++ = 10-100 fold, +++ = ≥ 100 fold, ++++ ≥1000 Example 19 - LNA-modified mRNA and saRNA demonstrate enhanced protein expression in Human Skin Explants Post-operative residual human skin tissue was used to assess the impact of LNA- modified RNA in a more human-relevant model with longer viability than cell culture. This established model is particularly relevant due to its preserved innate sensing profile, which can mimic the replication impediments observed clinically. The processed samples were injected with 2.5 μg of LNP formulated RNA and cultured for 21 days with periodic imaging (Figure 23). The selected conditions for the experiment were, for both mRNA and saRNA: Unmodified, as a control; m1Ψ, as a reference for the current standard use in the clinic; 90% LNA-GTP; or 90% LNA-ATP -CTP and -UTP (90% LNA-ACU), and PBS as a negative control. Expression for all the saRNA samples peaked at d3, and the unmodified and m1Ψ returned to baseline by d14. The locked saRNA samples (90% LNA-GTP and 90% LNA-ACU) presented higher expression than the unmodified and m1Ψ counterparts with detectable expression out to d21 (Figure 23a). For all mRNA constructs expression also peaked at d3, with the unmodified and m1Ψ modified mRNA returning to baseline by d14, while the locked mRNA samples (90% LNA-GTP and 90% LNA-ACU) presented higher expression than the unmodified and m1Ψ counterparts with detectable expression observed at d14 (Figure 23b). Assessment of expression by calculating expression by Area Under the Curve (AUC) showed increasing expression in the following order: UnModified saRNA < unmodified mRNA < m1Ψ mRNA < m1Ψ saRNA < 90% LNA-GTP mRNA < 90% LNA-ACU mRNA < 90% LNA-GTP saRNA < 90% LNA-ACU saRNA (Figure 23c). These data indicate that LNA modified RNA increased both the magnitude and duration of expression in ex-vivo human skin explants, where expression of LNA modified saRNA was greater than mRNA. Example 20 - LNA-modified mRNA and saRNA demonstrate enhanced protein expression in vivo in BALB/C Mice
Although the skin explants are a highly model, their ex vivo nature limits the length of expression to 21 days. Hence, expression in an in vivo model was also tested to determine whether the results translated in this more complex system.6–8- week-old BALB/C female mice were selected for this experiment, with 5 mice per group to assess comparative in vivo expression of unmodified mRNA, m1Ψ mRNA, 90% LNA- ACU mRNA, together with unmodified saRNA and 90% LNA-ACU (90% LNA-ATP - CTP and -UTP) saRNA. Each mouse received 2.5 μg of LNP-formulated RNA IM into each hind leg, to a final dose of 5 μg per mice, to enable 2 technical replicates from each animal. Luciferase expression (a marker of protein expression) in mice was quantified on days 1, 3, 7, 14, 21, 31 and 45 post-injection, following luciferin administration (Figure 24). All mRNA conditions peaked at 24h, with locked modified mRNA demonstrating a 1.5-log higher expression than m1Ψ and 2-log higher expression than unmodified mRNA at 24h (Figure 24a). Unmodified and m1Ψ mRNA expression was undetectable by d7 while expression of locked mRNA went out to d14, twice that of unmodified mRNA. Expression of unmodified saRNA peaked on d3 and decreased to minimal expression by d14; however, locked saRNA peaked at 24h with 2.5-log increased expression over the unmodified control, by d14 continued to express 2 logs higher than the canonical unmodified saRNA returning to baseline by d45. Analysis of expression by AUC (Figure 24b) indicated significantly increased expression by locked modified saRNA followed by locked mRNA> m1Ψ mRNA> Unmodified saRNA> Unmodified mRNA. Weight loss was monitored as a reporter of reactogenicity to the injection (Figure 24d). At 72h pi, from lowest to highest drop in weight loss was in the following order: mRNA locked< mRNA m1Ψ< VEEV locked< mRNA Unmod< VEEV Unmod. These data indicate that LNA modified RNA increases both the magnitude and duration of expression in vivo in mice, where expression of LNA modified saRNA was greater than mRNA. Example 21 - both LNA-modified mRNA and saRNA modulate expression of inflammatory cytokines in vivo in BALB/C Mice Mice were bled at baseline and 4h post administration of unmodified and modified mRNA and saRNA according to the conditions outlined in the example above, to determine systemic changes to the innate cytokine levels induced by the different RNAs, assessed using a serum MSD assay (Figure 25). A similar panel to the human MSD was selected for the mice, comprised of IFN proteins, inflammatory markers, chemoattractants and IFN-γ inducible genes. For mRNA, both m1Ψ and locked modified mRNA showed significantly reduced expression levels than unmodified
mRNA for IFN-α, -β and -γ, IP-10 and while only locked mRNA displayed significantly reduced levels for IL-6, KC, MIP-1α and MIP-1β compared to unmodified mRNA. For saRNA, locked modified saRNA samples showed significantly reduced IFN- α, IP-10 and MIP-1β levels relative to the unmodified saRNA, while no significant changes were observed for any of the other analytes. No significant difference was observed across any sample for IL-12p70. Overall, locked mRNA resulted in the lowest innate activation, followed by m1Ψ mRNA and locked saRNA, while unmodified mRNA and saRNA triggered the highest cytokine levels. These data indicate that LNA modification can reduce the expression of cytokines and chemokines induced by mRNA and saRNA injection in vivo. Summary The invention involves the incorporation of locked nucleic acids (LNA) into RNA sequences of greater than 25 nucleotides (NTPs), by in vitro transcription. It is demonstrated for the first time that mRNA containing LNA-NTPs can be translated by cellular ribosomes to generate functional proteins, and that the incorporation of LNA- NTPs both increases the level and duration of protein expression in transduced cells. LNAs are a modified version of RNA nucleotides where the ribose moiety is modified by a bridge between the 2’ oxygen and 4’ carbon. They are also referred to as “bridged nucleic acids” (BNA) or inaccessible RNA. Locking the ribose reduces the conformational flexibility of the ribose, increasing the local organization of the phosphate backbone and ultimately increases stability and potential recognition by RNA binding proteins. Previous RNA vaccines and therapeutics have used other synthetic modified versions of RNA, notably methyl-pseudo uridine (UTP), in order to reduce triggering of innate pathways that could restrict the expression of encoded proteins, rendering the RNA ineffective with respect to therapeutic or vaccine indications. The invention described herein demonstrates that different combinations of LNA-NTPs can also modulate the innate cytokine response to naked unmodified RNA induced when delivered into the cytoplasm of cells. A further surprising advantage is the discovery that the generation of RNA containing locked nucleotides by in vitro transcription is promoted by high levels of magnesium acetate, ideally greater than 20 mM, optimally greater than 40 mM. This opens the
possibility for enzymatic generation of sequence of any size using locked nucleotides. Important inventive features include: ^ Incorporation of LNAs into RNA sequences >25 NTPs by in vitro transcription; ^ Translation of mRNA containing LNA-NTPs into functional proteins; ^ LNA incorporation increases the level and duration of protein expression in transduced cells; ^ Combinations of LNA-NTPs that modulate the innate immune response; ^ Enzymatic synthesis of RNA containing LNAs is promoted by magnesium acetate. The inventors have therefore demonstrated that locked NTPs, where the furanose ring is modified by a bridge between the 2’- and the 4’-carbons, in particular 2’-O,4’- methylene-d-ribofuranosyl nucleotides, can be used to increase (i) expression and/or translation of an RNA molecule comprising the one or more modified LNA-NTP; (ii) enhancing the stability of an RNA molecule comprising the one or more modified LNA- NTP; and/or (iii) reducing the activation of innate sensing, interferon generation and/or degradation of an RNA molecule comprising the one or more LNA-NTP. Conclusions The invention involves the use of LNA-RNA, specifically mRNA, saRNA, circular RNA and non-coding RNA, with a proportion of nucleotides being LNA-NTPs. The mRNA and saRNA sequence are of a sizeable length (>100bp) and wild-type nucleotides are at least partially substituted by LNA-NTPs. The modified RNAs are synthesised via in vitro transcription, and the reaction mixture optimally includes high concentrations of magnesium ions (i.e. >20mM magnesium ions, ideally 75nM magnesium acetate, alternatively 40mM magnesium chloride) and high concentrations of nucleotides (i.e. >10mM nucleotides). The inventors have shown that magnesium ions presented in magnesium acetate results in enhanced expression and incorporation of the LNA-NTPs. Targeted partial substitution of wild-type GTP, ATP, CTP UTP and/or TTP with respective LNA-NTP led to enhanced expression and stability of modified mRNA or saRNA, by ≤100-fold, in interferon-responsive competent cells. The inventors have also shown that targeted
partial substitution of wild-type GTP, UTP and/or TTP with respective LNA- NTP was able to suppress induction of innate cytokine response to the RNA itself. References 1. Obika, Satoshi; Nanbu, Daishu; Hari, Yoshiyuki; Morio, Ken-ichiro; In, Yasuko; Ishida, Toshimasa; Imanishi, Takeshi. Synthesis of 2′-O,4′-C-methyleneuridine and -cytidine. Novel bicyclic nucleosides having a fixed C3, -endo sugar puckering. Tetrahedron Letters.1997, 38: 8735–8738. doi:10.1016/S0040- 4039(97)10322-7. 2. Orum, Miriam Frieden and Henrik. Locked Nucleic Acid Holds Promise in the Treatment of Cancer. Current Pharmaceutical Design.2008: 14: 1138–1142. doi:10.2174/138161208784246234. 3. Eichert, A. et al. The crystal structure of an ‘All Locked’ nucleic acid duplex. Nucleic Acids Res.2010: 38, 6729–6736. doi: 10.1093/nar/gkq505. 4. Förster C, Eichert A, Oberthür D, Betzel C, Geßner R, Nitsche A, Fürste JP. Features of "All LNA" Duplexes Showing a New Type of Nucleic Acid Geometry. J Nucleic Acids.2012;2012:156035. doi: 10.1155/2012/156035. 5. Yildirim I, Kierzek E, Kierzek R, Schatz GC. Interplay of LNA and 2'-O-methyl RNA in the structure and thermodynamics of RNA hybrid systems: a molecular dynamics study using the revised AMBER force field and comparison with experimental results. J Phys Chem B.2014;118:14177-87. doi: 10.1021/jp506703g. 6. Hull C, Szewcyk C, St John PM. Effects of locked nucleic acid substitutions on the stability of oligonucleotide hairpins. Nucleosides Nucleotides Nucleic Acids. 2012;31:28-41. doi: 10.1080/15257770.2011.639826. 7. Hughesman, C.B. et al. Role of the heat capacity change in understanding and modeling melting thermodynamics of complementary duplexes containing standard and nucleobase-modified LNA. Biochemistry 2011:50, 5354–5368 8. Liczner C, Duke K, Juneau G, Egli M, Wilds CJ. Beyond ribose and phosphate: Selected nucleic acid modifications for structure-function investigations and therapeutic applications. Beilstein J Org Chem.2021;17:908-931. doi: 10.3762/bjoc.17.76. 9. Soler-Bistué A, Zorreguieta A, Tolmasky ME. Bridged Nucleic Acids Reloaded. Molecules.2019;24:2297. doi: 10.3390/molecules24122297. 10. Mikkola S, Lönnberg T, Lönnberg H. Phosphodiester models for cleavage of nucleic acids. Beilstein J Org Chem.2018;14:803-837. doi: 10.3762/bjoc.14.68. 11. Zhang K, Hodge J, Chatterjee A, Moon TS, Parker KM. Duplex Structure of Double-Stranded RNA Provides Stability against Hydrolysis Relative to Single- Stranded RNA. Environ Sci Technol.2021;55:8045-8053. doi: 10.1021/acs.est.1c01255. 12. Goodchild, A., Nopper, N., King, A., Doan, T., Tanudji, M., Arndt, G. M., et al. Sequence determinants of innate immune activation by short interfering RNAs. BMC Immunology, 2009: 10, 40. DOI: 10.1186/1471-2172-10-40 13. Hornung, V., Guenthner-Biller, M., Bourquin, C., Ablasser, A., Schlee, M., Uematsu, S., et al. Sequence-specific potent induction of ifn-alpha by short interfering RNA in plasmacytoid dendritic cells through TLR7. Nature Medicine, 2005: 11, 263–270. doi: 10.1038/nm1191 14. Hong, J., Huang, Y., Li, J., Yi, F., Zheng, J., Huang, H., et al. Comprehensive analysis of sequence-specific stability of siRNA. The FASEB Journal, 2010: 24, 4844– 4855. doi: 10.1096/fj.09-142398.
15. Chernikov IV, Vlassov VV, EL. Current development of siRNA bioconjugates: from research to the clinic. Front Pharmacol.2019;10:444. DOI: 10.3389/fphar.2019.00444 16. Elmén J, Thonberg H, Ljungberg K, et al. Locked nucleic acid (LNA) mediated improvements in siRNA stability and functionality. Nucleic Acids Res. 2005;33(1):439–447. DOI:10.1093/nar/gki193. 17. Kurreck, J. Design of antisense oligonucleotides stabilized by locked nucleic acids. Nucleic Acids Research.2002: 30: 1911–1918. doi:10.1093/nar/30.9.1911. 18. Frieden, M. Expanding the design horizon of antisense oligonucleotides with alpha-L-LNA. Nucleic Acids Research.2003: 31: 6365–6372. DOI: 10.1093/nar/gkg820. 19. Frieden, Miriam; Hansen, Henrik F.; Koch, Troels. Nuclease Stability of LNA Oligonucleotides and LNA-DNA Chimeras. Nucleosides, Nucleotides and Nucleic Acids.2003: 22: 1041–1043. DOI: 10.1081/NCN-120022731 20. Morita, K.; Hasegawa, C.; Kaneko, M.; Tsutsumi, S.; Sone, J.; Ishikawa, T.; Imanishi, T.; Koizumi, M.2'-O, 4'-C-Ethylene-bridged nucleic acids (ENA) with nuclease-resistance and high affinity for RNA. Nucleic Acids Symposium Series. 2001: 1: 241–242. DOI: 10.1093/nass/1.1.241. 21. Lundin KE, Højland T, Hansen BR, Persson R, Bramsen JB, Kjems J, Koch T, Wengel J, Smith CI. Biological activity and biotechnological aspects of locked nucleic acids. Adv Genet.2013;82:47-107. doi: 10.1016/B978-0-12-407676- 1.00002-0. 22. Veedu RN, Vester B, Wengel J. Polymerase chain reaction and transcription using locked nucleic acid nucleotide triphosphates. J Am Chem Soc.2008 Jul 2;130(26):8124-5. doi: 10.1021/ja801389n. 23. Kore, A. R., Hodeib, M., & Hu, Z. Chemical synthesis of LNA-mctp and its application for microRNA detection. Nucleosides, Nucleotides & Nucleic Acids, 2008: 27, 1–17. doi: 10.1080/15257770701571693. 24. Morais P, Adachi H, Yu YT. The Critical Contribution of Pseudouridine to mRNA COVID-19 Vaccines. Front Cell Dev Biol.2021;9:789427. doi: 10.3389/fcell.2021.789427. 25. Karik K, Buckstein M, Ni H, Weissman D. Suppression of RNA recognition by Toll-like receptors: the impact of nucleoside modification and the evolutionary origin of RNA. Immunity.2005;23:165-75. doi: 10.1016/j.immuni.2005.06.008. 26. Andries O, Mc Cafferty S, De Smedt SC, Weiss R, Sanders NN, Kitada T. N(1)- methylpseudouridine-incorporated mRNA outperforms pseudouridine- incorporated mRNA by providing enhanced protein expression and reduced immunogenicity in mammalian cell lines and mice. J Control Release. 2015;217:337-44. doi: 10.1016/j.jconrel.2015.08.051. 27. Mu X, Greenwald E, Ahmad S, Hur S. An origin of the immunogenicity of in vitro transcribed RNA. Nucleic Acids Res.2018 Jun 1;46(10):5239-5249. 28. Damian L, Marty-Detraves C, Winterhalter M, Fournier D, Paquereau L. Single- strand DNA translation initiation step analyzed by Isothermal Titration Calorimetry. Biochem Biophys Res Commun.2009 Jul 31;385(3):296-301. doi: 10.1016/j.bbrc.2009.05.044. 29. McCown PJ, Ruszkowska A, Kunkler CN, Breger K, Hulewicz JP, Wang MC, Springer NA, Brown JA. Naturally occurring modified ribonucleosides. Wiley Interdiscip Rev RNA.2020 Sep;11(5):e1595. doi: 10.1002/wrna.1595. 30. Lee JS, Woodsworth ML, Latimer LJ, Morgan AR. Poly(pyrimidine) . poly(purine) synthetic DNAs containing 5-methylcytosine form stable triplexes at neutral pH. Nucleic Acids Res.1984;12(16):6603-14. doi: 10.1093/nar/12.16.6603.
Bretscher MS,. Ribosome the mode of action of neomycin in the direct translation of single-stranded fd DNA. Cold Spring Harb Symp Quant Biol.1969;34:651-3. doi: 10.1101/sqb.1969.034.01.075.