Atty. Dkt. No.: 115872-2919 METHODS FOR ENHANCING THE EFFICACY OF RNAI THERAPY BY TARGETING ALAS1/ALAS2 CROSS-REFERENCE TO RELATED APPLICATIONS [0001] This application claims the benefit of and priority to U.S. Provisional Patent Application No.63/437,575, filed January 6, 2023, and U.S. Provisional Patent Application No. 63/461,700, filed April 25, 2023, the contents of which are incorporated herein by reference in their entireties. TECHNICAL FIELD [0002] The present technology relates to methods for enhancing the efficacy of RNAi therapy (e.g., therapeutic agents including siRNAs or miRNAs that target disease-inducing genes) in a subject in need thereof. Also disclosed herein are compositions for reducing the expression and/or activity of ALAS1 and/or ALAS2 and methods of using the same as RNAi adjuvants in vivo. GOVERNMENT SUPPORT [0003] This invention was made with government support under GM083300 awarded by the National Institutes of Health. The government has certain rights in the invention.”. BACKGROUND [0004] The following description of the background of the present technology is provided simply as an aid in understanding the present technology and is not admitted to describe or constitute prior art to the present technology. [0005] RNA interference (RNAi) and microRNA (miRNA) pathways are related strategies for small RNA mediated-silencing of complementary targets. In mammals, stepwise cleavage of hairpin transcripts by the nuclear Drosha/DGCR8 ("Microprocessor") complex and cytoplasmic Dicer yields ~22 nucleotide (nt) miRNAs. These are bound by any of four Argonaute effectors (Ago1-4), which associate with the adaptor GW182 (TNRC6) to repress targets with pairing to the miRNA 5' ends (the seed region, nts 2-8). Endogenous miRNAs, as well as synthetic small -1- 4892-3380-3674.1
Atty. Dkt. No.: 115872-2919 interfering RNAs (siRNAs) that load into Ago2, the sole mammalian Ago with robust enzymatic activity, can guide the cleavage of highly complementary targets in a GW182-independent fashion. The recognition of the RNAi and miRNA pathways propelled new frontiers in the understanding of gene regulatory mechanisms, and provided crucial strategies to silence arbitrary genes of choice (i.e., "knockdown" studies). [0006] Given its broad utility in experimental science, RNAi has attracted great interest as a novel molecular strategy to silence disease-inducing genes. However, appropriate cellular delivery and effective in vivo silencing of siRNAs have proven challenging. Numerous other RNAi drugs are under development to treat an array of hepatic and extra-hepatic diseases, including cancer, Type 2 diabetes, and Alzheimer’s disease. Nevertheless, obtaining effective silencing remains a central hurdle. SUMMARY OF THE PRESENT TECHNOLOGY [0007] In one aspect, the present disclosure provides a method for enhancing efficacy of RNAi therapy in a subject in need thereof, comprising administering to the subject an effective amount of at least one ALAS1-specific inhibitory nucleic acid as disclosed herein and/or at least one ALAS2-specific inhibitory nucleic acid as disclosed herein, wherein the at least one ALAS1- specific inhibitory nucleic acid and/or at least one ALAS2-specific inhibitory nucleic acid inhibits expression levels or activity of 5’-aminolevulinic acid synthase (ALAS) polypeptide in the subject; and administering to the subject an effective amount of at least one RNAi agent that inhibits expression of a disease-inducing gene, wherein the disease-inducing gene is not an ALAS gene. The at least one ALAS1-specific inhibitory nucleic acid and/or at least one ALAS2-specific inhibitory nucleic acid and the at least one RNAi agent may be administered separately, simultaneously or sequentially. Additionally or alternatively, in some embodiments, the at least one ALAS1-specific inhibitory nucleic acid and/or at least one ALAS2-specific inhibitory nucleic acid is a siRNA, a shRNA, an antisense oligonucleotide, or a sgRNA. In certain embodiments, the at least one ALAS1-specific inhibitory nucleic acid comprises a nucleic acid sequence selected from the group consisting of: SEQ ID NO: 8, SEQ ID NO: 9, -2- 4892-3380-3674.1
Atty. Dkt. No.: 115872-2919 SEQ ID NO: 10, SEQ ID NO: 11, SEQ ID NO: 95, SEQ ID NO: 96, or a complement thereof or comprises givosiran. [0008] Additionally or alternatively, in some embodiments of the methods disclosed herein, the at least one RNAi agent comprises a siRNA or a miRNA. In certain embodiments, the miRNA is a tumor-suppressor miRNA. Examples of tumor-suppressor miRNAs include, but are not limited to let-7, miR-15/16, miR-19, miR-34, miR-34a, miR-140, miR-148a, miR-340, miR- 133a-5p, miR-34b-3p, miR-296, miR-188-5p, miR-1291, miR-591, miR-30, miR-188-5p, miR- 34b/c, miR-203, miR141, miR-320, miR-429, and miR-200. In some embodiments, the tumor- suppressor miRNA is a member of a miR family selected from among miR-29 family, let-7 family, mir-30 family and mir-34/449 family. [0009] In any of preceding embodiments of the methods disclosed herein, the at least one ALAS1-specific inhibitory nucleic acid and/or at least one ALAS2-specific inhibitory nucleic acid is administered orally, topically, intranasally, systemically, intravenously, subcutaneously, intraperitoneally, intradermally, intraocularly, iontophoretically, transmucosally, or intramuscularly. Additionally or alternatively, in some embodiments, the at least one RNAi agent is administered orally, topically, intranasally, systemically, intravenously, subcutaneously, intraperitoneally, intradermally, intraocularly, iontophoretically, transmucosally, or intramuscularly. [0010] Additionally or alternatively, in some embodiments of the methods disclosed herein, administration of the at least one RNAi agent comprises administering an effective amount of an expression vector comprising a nucleic acid sequence encoding the at least one RNAi agent. In certain embodiments, the nucleic acid sequence encoding the at least one RNAi agent is operably linked to a constitutive promoter, an inducible promoter, a tissue-specific promoter or an ubiquitous promoter. [0011] Additionally or alternatively, in certain embodiments of the methods disclosed herein, administration of the at least one ALAS1-specific inhibitory nucleic acid and/or at least one ALAS2-specific inhibitory nucleic acid comprises administering an effective amount of an expression vector comprising a nucleic acid sequence encoding the at least one ALAS1-specific -3- 4892-3380-3674.1
Atty. Dkt. No.: 115872-2919 inhibitory nucleic acid or the at least one ALAS2-specific inhibitory nucleic acid. In some embodiments, the nucleic acid sequence encoding the at least one ALAS1-specific inhibitory nucleic acid or the at least one ALAS2-specific inhibitory nucleic acid is operably linked to a constitutive promoter, an inducible promoter, a tissue-specific promoter or an ubiquitous promoter. [0012] In any and all embodiments of the methods disclosed herein, the at least one RNAi agent, the at least one ALAS1-specific inhibitory nucleic acid and/or the at least one ALAS2- specific inhibitory nucleic acid is conjugated to a ligand selected from among a protein, a carbohydrate, a lipid and a drug. Examples of suitable ligands include, but are not limited to human serum albumin (HSA), low-density lipoprotein (LDL), globulin, a dextran, pullulan, chitin, chitosan, inulin, cyclodextrin, hyaluronic acid, lectin, thyrotropin, melanotropin, lectin, glycoprotein, surfactant protein A, mucin carbohydrate, multivalent lactose, multivalent galactose, N-acetyl-galactosamine, N-acetyl-glucosamine multivalent mannose, multivalent fucose, glycosylated polyaminoacids, multivalent galactose, transferrin, bisphosphonate, polyglutamate, polyaspartate, cholesterol, a steroid, bile acid, folate, vitamin B12, biotin, a RGD peptide or a RGD peptide mimetic. [0013] In any of the above embodiments of the methods disclosed herein, the at least one RNAi agent, the at least one ALAS1-specific inhibitory nucleic acid and/or the at least one ALAS2-specific inhibitory nucleic acid is formulated as a lipid nanoparticle (LNP) or a stable nucleic acid lipid particle (SNALP). [0014] Additionally or alternatively, in some embodiments of the methods disclosed herein, the at least one RNAi agent comprises one or more of patisiran, lumasiran, vutrisiran, inclisiran, cemdisiran, zilebesiran, ALN-XDH, ALN-APP, ALN-HSD, and AB-729. [0015] In any and all embodiments of the methods disclosed herein, the subject suffers from, is diagnosed with, or is at risk for Human papillomavirus (HPV), age-related macular degeneration, Alzheimer’s disease, depression, Huntington’s disease, spinocerebral ataxia, ALS, encephalitis, SARS, influenza, herpes, glioblastoma, prostate cancer, adenocarcinoma, irritable bowel syndrome, RSV, hepatitis B, nonalcoholic steatohepatitis, hypertension, gout, paroxysmal -4- 4892-3380-3674.1
Atty. Dkt. No.: 115872-2919 nocturnal hemoglobinuria, polyneuropathy (sensorimotor and autonomic neuropathy), amyloidosis, hereditary transthyretin-mediated amyloidosis, wild-type transthyretin-mediated amyloidosis (ATTRwt), primary hyperoxaluria type 1 (PH1), atherosclerotic cardiovascular disease, rheumatoid arthritis, hypercholesterolemia, pancreatic ductal adenocarcinoma, relapsed or refractory B cell non-Hodgkin lymphoma, dry eye disease, open angle glaucoma, or acute kidney injury. In some embodiments, the subject is human. [0016] Additionally or alternatively, in some embodiments of the methods disclosed herein, the disease-inducing gene is an oncogene, a glycoprotein, an enzyme, a proton-coupled folate transporter, a transport protein, a hormone, or a viral protein. In some embodiments, the disease- inducing gene encodes Transthyretin, glycolate oxidase, PCSK9, Complement component 5, angiotensinogen (AGT), xanthine dehydrogenase (XDH), amyloid precursor protein (APP), or 17β-Hydroxysteroid dehydrogenase type 13. The disease-inducing gene may be expressed in one or more cell types, including but not limited to, hepatocytes, neurons, astrocytes, microglial cells, retinal pigment epithelial cells, pancreatic ductal cells, blood cells, alveolar cells, tumor cells, intestinal cells, and vaginal epithelial cells. BRIEF DESCRIPTION OF THE DRAWINGS [0017] FIG.1 shows the core miRNA/RNAi pathway in human cells. Canonical primary miRNA (pri-miRNA) transcripts contain a hairpin that is cleaved by the nuclear RNase III enzyme Drosha and its partner DGCR8, which releases a pre-miRNA hairpin that is cleaved by the cytoplasmic RNase III enzyme Dicer. The small RNA duplex is loaded into one of the four Argonaute (proteins) and guides it to complementary targets for regulation. Typically, endogenous mRNA targets pair with short ~7nt complements to the 5' ends of miRNAs (the "seed region"). If the small RNA is highly complementary to target, and resides in the Ago2 complex, the target can be cleaved ("Slicing"). Non-canonical substrates and even synthetic small interfering RNA duplexes (siRNAs) can also enter Ago complexes and program gene suppression. [0018] FIG.2 shows the heme biosynthetic pathway. Heme is generated via a cellular pathway consisting of eight enzymes, designated by ovals, several of which function within -5- 4892-3380-3674.1
Atty. Dkt. No.: 115872-2919 mitochondria. ALAS1 catalyzes the first step in the process, and occupies a pivotal position in the pathway that is subject to feedback regulation. [0019] FIG.3 demonstrates that heme controls microprocessor activity in pri-miRNA cleavage. (Left) Sequence and structural features of typical canonical miRNA hairpins. (Middle) DGCR8 binds hemin, which stabilizes the DGCR8 dimer and helps orient the position of Drosha on the pri-miRNA hairpin. (Right) In the absence of heme, both the activity and accuracy of pri- miRNA cleavage are compromised. [0020] FIG.4 shows the expression patterns of human ALAS family genes. ALAS1 is ubiquitously expressed across a broad panel of human cell lines, while ALAS1 is specific to cell lines of erythroid origin. [0021] FIGs.5A-5C show the generation and validation of HEK293T knockout cell lines for core heme biosynthetic factors. FIG.5A: Western blot showing that independent ALAS1 knockout cell lines, generated with different sgRNA sequences, lack ALAS1 protein. FIG.5B: rt-PCR shows that erythroid-specific paralog ALAS2 is not induced upon the loss of ALAS1. FIG.5C: Western blot validation of ALAS1 and CPOX knockout cell lines. These proteins are absent from the respective knockout backgrounds, but CPOX knockout also induces high levels of ALAS1 protein. [0022] FIGs.6A-6C show the validation of defective pri-miRNA processing in heme pathway knockout cells. FIG.6A: Scheme for pri-miRNA processing sensor. The 3' UTR of a luciferase expression construct contains pri-mir-375. If the miRNA is not cleaved, luciferase protein can be translated. If the miRNA hairpin is cleaved, then the mRNA lacking the poly-A tail (A
n) will be degraded. FIG.6B: Activity of the pri-miRNA processing sensor in control and heme pathway knockout cells, in basal media (left) and heme-depleted media (right). The pri- miRNA processing sensor increases in both ALAS1 and CPOX knockout cells, but only in heme- depleted conditions. FIG.6C: Endogenous pri-miRNA transcript levels were not altered in ALAS1 knockout cells. [0023] FIGs.7A-7B demonstrate that ALAS1 knockout increases mature miRNA levels. FIG.7A: Northern blotting for several miRNAs and control 5S rRNA shows increased miRNA -6- 4892-3380-3674.1
Atty. Dkt. No.: 115872-2919 levels in independent ALAS1 knockout cells compared to control HEK293T cells. FIG.7B: Ago2 was immunoprecipitated (IP) and associated RNAs were analyzed by Northern blotting. Multiple miRNAs were increased in Ago2-IP from ALAS1 knockout cells. [0024] FIG.8 demonstrates that ALAS1 knockout globally increases mature miRNA levels. Small RNAs were cloned from control and ALAS1 knockout HEK293T cells using external spike-in normalization. Each dot represents a miRNA species, which are globally increased in ALAS1 knockout cells. [0025] FIG.9 demonstrates that ALAS1 knockout does not affect miRNA terminal structures. Normal miRNAs carry 5' monophosphate (PO
4) and a free 3' hydroxyl group (OH); abnormal miRNAs might lack 5'-PO
4 or potentially gain 3' methylation (CH
3). These terminal structures can be interrogated by chemical treatments such as calf intestinal phosphatase (CIP) to remove 5'-PO
4, or ß-elimination, which alters the composition of free 3' ends but is blocked by methylation. These treatments decrease or increase small RNA mobility, respectively. The properties of miRNAs isolated from ALAS1 knockout cells are similar to control wildtype (WT) cells. [0026] FIGs.10A-10C demonstrate that ALAS1 knockout enhances RISC assembly. FIG.10A: Scheme to interrogate maturation of the RNA induced silencing complex (RISC) in vitro. The size shift from duplex-loaded Ago2 to single-stranded Ago2 can be monitored on native gels, with the latter migrating faster than the former. FIG.10B: Time course studies show that RISC maturation is accelerated using materials from independent ALAS1 knockout cells. FIG.10C: Quantification of the data in FIG.10B. [0027] FIGs.11A-11C demonstrate that ALAS1 knockout enhances the accumulation of Ago2-GW182 complexes in cells. FIG.11A: GW182 (also known as TNRC6) is an essential cofactor for Ago proteins in post-transcriptional silencing via target mRNA 5' and 3' termini. FIG.11B: Co-immunoprecipitation assays show that more Ago2 is associated with GFP-GW182 in ALAS1 knockout cells, compared to parental HEK293T cells. ALAS1 protein is not specifically associated with GW182. FIG.11C: Quantification of three experiments of the type shown in FIG.11B. -7- 4892-3380-3674.1
Atty. Dkt. No.: 115872-2919 [0028] FIGs.12A-12B demonstrate that core heme biosynthetic factor CPOX does not repress miRNA levels or activity. FIG.12A: Northern blots show that mature miRNAs are increased in ALAS1 knockout and ALAS1/CPOX double knockout cells, but not in CPOX knockout cells. FIG.12B: Functional miRNA sensor assays show enhanced miRNA-mediated repression in ALAS1 knockout, while CPOX knockout cells show a moderate opposite trend of decreased miRNA efficacy. The ALAS1/CPOX double knockout cells exhibit epistasis for the ALAS1 phenotype, i.e., enhanced miRNA activity. [0029] FIGs.13A-13B demonstrate that core heme biosynthetic factor CPOX does not repress RISC formation. FIG.13A: In vitro RISC maturation assays were performed as in FIG.10A. Only ALAS1 knockout and ALAS1/CPOX double knockout cells exhibit enhanced RISC maturation; CPOX knockouts are similar to wildtype. (B) Quantification of B. Note that CPOX knockouts exhibit slightly less efficient RISC maturation, and the ALAS1/CPOX double knockout cells exhibit epistasis for the ALAS1 phenotype, i.e., enhanced RISC assembly. [0030] FIG.14 shows a comparison of canonical miRNA and RNase III-independent miRNA pathways. (Left) Canonical miRNA biogenesis involves stepwise cleavages of hairpin substrates via Drosha and Dicer RNase III enzymes. (Right) Drosha cleavage can be substituted by RNase Z, which is normally involved in tRNA biogenesis. Dicer cleavage can be bypassed by the pre-mir-451 hairpin, which is too short to be cleaved by Dicer, and is instead directly loaded in Ago2, whereby slicing of its 3' end enables resection into a mature, active, miR-451 species. Thus, tRNA-mir-451 permits biogenesis steps downstream of RNase III cleavages to be interrogated. [0031] FIGs.15A-15B demonstrate RNase III-independent genetic sensor of miRNA activity. FIG.15A: Dual miRNA-reporter transgene contains a tRNA-mir-451 construct, and an RFP sensor that is repressed by miR-451. FIG.15A: A stable cell line bearing the dual miRNA- reporter transgene was selected for intermediate RFP fluorescence. CRISPR/Cas9 editing can be performed in this cell line, and FACS can be used to separate out cells with lower RFP, which may reflect enhanced miRNA activity. -8- 4892-3380-3674.1
Atty. Dkt. No.: 115872-2919 [0032] FIGs.16A-16B demonstrate that ALAS1 acts independently of other heme biosynthetic factors to repress the miRNA pathway. FIG.16A: Western blot validation of heterogenous knockout cells for 5 core heme enzymes (FIG.2). Independent ALAS1 sgRNAs yield decreased ALAS1 protein. We infer that the remaining ALAS1 protein reflects that some cells are not ALAS1 null in this heterogenous editing setting (compared to complete absence in single-cell cloned ALAS1 knockout cells, e.g., FIG.5A). Reciprocally, the efficacy of knockouts for all heme biosynthetic enzymes downstream of ALAS1 all induce higher levels of ALAS1 protein, due to feedback regulation. FIG.16B: Using these heterogenously edited cells, a population of cells with lower RFP (increased miRNA activity) was obtained with independent ALAS1 sgRNAs, but 5 other sgRNAs for other heme biosynthetic enzymes did not phenocopy ALAS1 mutation. [0033] FIGs.17A-17B show a molecular strategy to assess Argonaute/miRNA/target complexes. FIG.17A: Ago2-CLEAR-CLIP protocol involves purification of the ternary Argonaute/miRNA/target complex, limited RNase digestion of target mRNA regions bound by Ago2, and ligation of miRNAs to target sequences. The ligation step is low efficiency, so that miRNA:target chimeric reads compromise a minority of unligated miRNA and target mRNA reads. FIG.17B: Autoradiograph demonstrating successful isolation of Ago2-miRNA-target complexes, which were isolated for library preparation. [0034] FIGs.18A-18C demonstrate that ALAS1 knockout enhances levels of endogenous Argonaute/miRNA/target complexes. FIG.18A: Genome browser view showing non-chimeric and miR-92a-3p-chimeric reads at the example locus SERTAD3. ALAS1 knockout results in higher Ago2-bound mRNA reads, as well as miR-92a-3p:mRNA chimeric reads, precisely at the location of a conserved miR-92-3p seed match in SERTAD3. FIG.18B: Independent biochemical assay demonstrating that levels of SERTAD3 transcript associated with Ago2 is increased in ALAS1 knockout cells. FIG.18C: Luciferase-SERTAD3 sensor assay showing that DICER-KO eliminates its repression, whereas ALAS1 knockout increases its repression. -9- 4892-3380-3674.1
Atty. Dkt. No.: 115872-2919 [0035] FIGs.19A-19C demonstrate global enhancement of endogenous Argonaute/miRNA/target complexes in ALAS1 knockout cells. FIG.19A: The levels of miRNA-target mRNA chimeric reads in Ago2 CLEAR-CLIP data are increased in ALAS1 knockout cells. FIG.19B: Plotting of individual loci shows that miRNAs and miRNA-target chimeric reads are broadly increased in ALAS1 knockout cells. FIG.19C: Coverage plots of target mRNA fragments at miRNA sites in Ago2 CLEAR-CLIP data shows globally
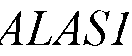
increased levels in ALAS1 knockout [0036] FIGs.20A-20D show the generation of Alas1-LoxP mouse line. FIG.20A: Basic structure of targeting vector. Long arm (LA), middle arm (MA), and short arm (SA) are denoted in red, green and blue, respectively. Triangles and crescents represent loxP and FRT sites, respectively. FIG.20B: Illustration of primers used to identify recombinant ES clones. FIG. 20C: Southern blot for PCR-identified positive clones 111, 181, 213, and 383 following Hind III digestion. The probed fragment in the targeted allele is 6.5 Kb, whereas it is 7.6 Kb in the wildtype allele. FIG.20D: Southern blot for PCR-identified positive clones 111, 181, 213, and 383 following Mfe I digestion. The probed fragment size within the targeted allele is 19.8 Kb, whereas it is 22.5 Kb for the wildtype allele. [0037] FIGs.21A-21E demonstrate that hepatocyte-specific Alas1/2-KO mice have essentially no hepatic ALAS activity. FIG.21A: PCR amplicons spanning Alas1 exons 3-6 show efficient exon 4-5 excision in liver (Lv) but not brain (Br), heart (Ht), or kidney (Kd) of Alas1-KO mice. FIG.21B: Representative western blot image using anti-ALAS1 antibody on murine liver homogenates. To confirm specificity of the antibody, T1T2 acute intermittent porphyria mice were challenged with the ALAS1-inducing drug phenobarbital (PB), either alone or following knockdown (KD) of Alas1 using siRNA (PB/KD). T1T2 mice are deficient in HMBS, the third heme biosynthetic enzyme, and as a result, markedly increase their hepatic ALAS1 expression when subjected to heme-consuming agents such as PB. FIG.21C: Relative Alas1 and Alas2 mRNA quantitated in whole livers of Cre- controls and Alas1-KO (1KO) mice. FIG.21D: ALAS activity in livers of Cre- controls, Alas1-KO (1KO) and Alas1/2-KO (1/2KO). LLD, lower limit of detection. FIG.21E: Representative Alas1/2-KO liver section stained using -10- 4892-3380-3674.1
Atty. Dkt. No.: 115872-2919 an anti-EGFP antibody to detect Cre expressing cells. Cre expression (represented by brown pigmentation) is abundant in hepatocytes but absent in non-hepatocytic cells, including portal vein (PV), bile duct (BD), hepatic artery (HA), and central veins (CV). Purple indicates nuclear staining. 100x magnification, 200 mM scale bar is at lower right corner. [0038] FIG.22 demonstrate that ALAS-deficient hepatocytes maintain near-normal heme content. Heme content and activities of representative hemoproteins, including cytochrome P450 (CYP)1A2, nitric oxide synthase (NOS), and catalase, determined in Cre- controls (C) and Alas1/2-KO (1/2KO) male hepatocytes isolated by differential centrifugation. [0039] FIGs.23A-23C demonstrate that ALAS-deficient hepatocytes exhibit global increase in mature miRNAs. FIG.23A: Northern blotting of hepatocyte RNA samples from control mice and Alas1/2-KO (A12KO) mice. The levels of multiple mature miRNAs are increased. FIG.23B: Quantification of independent Northern analyses. FIG.23C: Small RNA sequencing from control and Alas1/2-KO hepatocytes demonstrates a directional shift to increased miRNAs in mutants. [0040] FIGs.24A-24E demonstrate that ALAS deficient cells support increased silencing from miRNA and siRNA triggers. Silencing assays were conducted in ALAS1- KO HEK293T cells. FIG.24A: Scheme for expression of RNase III-independent tRNA-mir- 451, which represses a luciferase sensor bearing 4x miR-451 sites. FIG.24B: Silencing of the miR-451 sensor is increased in independent ALAS1-KO cells. FIG.24C: Scheme for repression of stable GFP transgene by synthetic GFP siRNA duplex. FIG.24D: Western blot analysis shows that, 24 hours after introduction of GFP siRNA (siGFP), GFP protein levels are suppressed more strongly in ALAS1-KO cells than in parental wild-type cells. FIG. 24E: Quantification of GFP protein depletion across three replicate experiments. [0041] FIG.25 demonstrates that ablation of ALAS enhances siRNA-mediated silencing in hepatocytes. Hepatocyte-specific Alas1/2KO male mice and sex- and age-matched Cre- control mice were administered a single extremely small dose (0.0001 mg/kg, s.c.) of GalNAc- conjugated siRNA targeting Slc46A1 at 48 h following the last TMX and/or Alas2-siRNA dose. Hepatocytes were isolated (denoted by the X mark) 24 h following administration of the -11- 4892-3380-3674.1
Atty. Dkt. No.: 115872-2919 Slc46A1-siRNA and assessed for Slc46A1 mRNA by qPCR. While Cre-hepatocytes had ~38% knockdown of Slc46A1 mRNA, Alas1/2KO hepatocytes achieved ~65% knockdown relative to levels in Cre- male hepatocytes that were not treated with Slc46A1-siRNA. p = 0.037 by one-way ANOVA with post-hoc analyses using Turkey’s multiple comparison test. DETAILED DESCRIPTION [0042] It is to be appreciated that certain aspects, modes, embodiments, variations and features of the present methods are described below in various levels of detail in order to provide a substantial understanding of the present technology. [0043] In practicing the present methods, many conventional techniques in molecular biology, protein biochemistry, cell biology, immunology, microbiology and recombinant DNA are used. See, e.g., Sambrook and Russell eds. (2001) Molecular Cloning: A Laboratory Manual, 3rd edition; the series Ausubel et al. eds. (2007) Current Protocols in Molecular Biology; the series Methods in Enzymology (Academic Press, Inc., N.Y.); MacPherson et al. (1991) PCR 1: A Practical Approach (IRL Press at Oxford University Press); MacPherson et al. (1995) PCR 2: A Practical Approach; Harlow and Lane eds. (1999) Antibodies, A Laboratory Manual; Freshney (2005) Culture of Animal Cells: A Manual of Basic Technique, 5th edition; Gait ed. (1984) Oligonucleotide Synthesis; U.S. Patent No.4,683,195; Hames and Higgins eds. (1984) Nucleic Acid Hybridization; Anderson (1999) Nucleic Acid Hybridization; Hames and Higgins eds. (1984) Transcription and Translation; Immobilized Cells and Enzymes (IRL Press (1986)); Perbal (1984) A Practical Guide to Molecular Cloning; Miller and Calos eds. (1987) Gene Transfer Vectors for Mammalian Cells (Cold Spring Harbor Laboratory); Makrides ed. (2003) Gene Transfer and Expression in Mammalian Cells; Mayer and Walker eds. (1987) Immunochemical Methods in Cell and Molecular Biology (Academic Press, London); and Herzenberg et al. eds (1996) Weir’s Handbook of Experimental Immunology. Methods to detect and measure levels of polypeptide gene expression products (i.e., gene translation level) are well- known in the art and include the use of polypeptide detection methods such as antibody detection and quantification techniques. (See also, Strachan & Read, Human Molecular Genetics, Second Edition. (John Wiley and Sons, Inc., NY, 1999)). -12- 4892-3380-3674.1
Atty. Dkt. No.: 115872-2919 [0044] The present technology relates generally to methods that harness Argonaute-mediated small RNA silencing. These include silencing by microRNAs (miRNAs) as well as by synthetic small interfering RNAs (siRNAs) that are the active species that program RNA interference (RNAi). Disclosed are data showing that ALAS1, the key regulatory enzyme in the heme biosynthesis pathway, has a heme-independent function to suppress late stages in the microRNA pathway, downstream of the central RNase III enzymes Drosha and Dicer. Further, loss of ALAS1 enhances the accumulation of small RNAs in functional Argonaute complexes. As demonstrated by the Examples herein, the RNAi adjuvant compositions of the present technology reduce the expression and/or activity of ALAS1 and/or ALAS2, which consequently enhances the overall efficacy of RNAi therapy (e.g., therapeutic agents including siRNAs or miRNAs that target disease-inducing genes) across various tissues. Definitions [0045] Unless defined otherwise, all technical and scientific terms used herein generally have the same meaning as commonly understood by one of ordinary skill in the art to which this technology belongs. As used in this specification and the appended claims, the singular forms “a”, “an” and “the” include plural referents unless the content clearly dictates otherwise. For example, reference to “a cell” includes a combination of two or more cells, and the like. Generally, the nomenclature used herein and the laboratory procedures in cell culture, molecular genetics, organic chemistry, analytical chemistry and nucleic acid chemistry and hybridization described below are those well-known and commonly employed in the art. [0046] As used herein, the term “about” in reference to a number is generally taken to include numbers that fall within a range of 1%, 5%, or 10% in either direction (greater than or less than) of the number unless otherwise stated or otherwise evident from the context (except where such number would be less than 0% or exceed 100% of a possible value). [0047] As used herein, the “administration” of an agent or drug to a subject includes any route of introducing or delivering to a subject a compound to perform its intended function. Administration can be carried out by any suitable route, including orally, intranasally, -13- 4892-3380-3674.1
Atty. Dkt. No.: 115872-2919 parenterally (intravenously, intramuscularly, intraperitoneally, or subcutaneously), or topically. Administration includes self-administration and the administration by another. [0048] The terms “blunt” or “blunt ended” as used herein in reference to a dsRNA mean that there are no unpaired nucleotides or nucleotide analogs at a given terminal end of a dsRNA, i.e., no nucleotide overhang. One or both ends of a dsRNA can be blunt. Where both ends of a dsRNA are blunt, the dsRNA is said to be blunt ended. To be clear, a “blunt ended” dsRNA is a dsRNA that is blunt at both ends, i.e., no nucleotide overhang at either end of the molecule. Most often such a molecule will be double-stranded over its entire length. [0049] The terms “complementary” or “complementarity” as used herein with reference to polynucleotides (i.e., a sequence of nucleotides such as an oligonucleotide or a target nucleic acid) refer to the base-pairing rules. The complement of a nucleic acid sequence as used herein refers to an oligonucleotide which, when aligned with the nucleic acid sequence such that the 5' end of one sequence is paired with the 3' end of the other, is in “antiparallel association.” For example, the sequence “5'-A-G-T-3'” is complementary to the sequence “3'-T-C-A-5.” Certain bases not commonly found in naturally-occurring nucleic acids may be included in the nucleic acids described herein. These include, for example, inosine, 7-deazaguanine, Locked Nucleic Acids (LNA), and Peptide Nucleic Acids (PNA). Complementarity need not be perfect; stable duplexes may contain mismatched base pairs, degenerative, or unmatched bases. Those skilled in the art of nucleic acid technology can determine duplex stability empirically considering a number of variables including, for example, the length of the oligonucleotide, base composition and sequence of the oligonucleotide, ionic strength and incidence of mismatched base pairs. A complementary sequence can also be an RNA sequence complementary to the DNA sequence or its complementary sequence, and can also be a cDNA. [0050] As used herein, a "control" is an alternative sample used in an experiment for comparison purpose. A control can be "positive" or "negative." For example, where the purpose of the experiment is to determine a correlation of the efficacy of a therapeutic agent for the treatment for a particular type of disease or condition, a positive control (a compound or -14- 4892-3380-3674.1
Atty. Dkt. No.: 115872-2919 composition known to exhibit the desired therapeutic effect) and a negative control (a subject or a sample that does not receive the therapy or receives a placebo) are typically employed. [0051] The term “double-stranded RNA” or “dsRNA,” as used herein, refers to an iRNA that includes an RNA molecule or complex of molecules having a hybridized duplex region that comprises two anti-parallel and substantially complementary nucleic acid strands, which will be referred to as having “sense” and “antisense” orientations with respect to a target RNA. The duplex region can be of any length that permits specific degradation of a desired target RNA, e.g., through a RISC pathway, but will typically range from 9 to 36 base pairs in length, e.g., 15- 30 base pairs in length. Considering a duplex between 9 and 36 base pairs, the duplex can be any length in this range, for example, 9, 10, 11, 12, 13, 14, 15, 16, 17, 18, 19, 20, 21, 22, 23, 24, 25, 26, 27, 28, 29, 30, 31, 32, 33, 34, 35, or 36 and any sub-range therein between, including, but not limited to 15-30 base pairs, 15-26 base pairs, 15-23 base pairs, 15-22 base pairs, 15-21 base pairs, 15-20 base pairs, 15-19 base pairs, 15-18 base pairs, 15-17 base pairs, 18-30 base pairs, 18-26 base pairs, 18-23 base pairs, 18-22 base pairs, 18-21 base pairs, 18-20 base pairs, 19-30 base pairs, 19-26 base pairs, 19-23 base pairs, 19-22 base pairs, 19-21 base pairs, 19-20 base pairs, 20-30 base pairs, 20-26 base pairs, 20-25 base pairs, 20-24 base pairs, 20-23 base pairs, 20-22 base pairs, 20-21 base pairs, 21-30 base pairs, 21-26 base pairs, 21-25 base pairs, 21-24 base pairs, 21-23 base pairs, or 21-22 base pairs. dsRNAs generated in the cell by processing with Dicer and similar enzymes are generally in the range of 19-22 base pairs in length. One strand of the duplex region of a dsDNA comprises a sequence that is substantially complementary to a region of a target RNA. The two strands forming the duplex structure can be from a single RNA molecule having at least one self-complementary region, or can be formed from two or more separate RNA molecules. Where the duplex region is formed from two strands of a single molecule, the molecule can have a duplex region separated by a single stranded chain of nucleotides (herein referred to as a “hairpin loop”) between the 3′-end of one strand and the 5′-end of the respective other strand forming the duplex structure. The hairpin loop can comprise at least one unpaired nucleotide; in some embodiments the hairpin loop can comprise at least 3, at least 4, at least 5, at least 6, at least 7, at least 8, at least 9, at least 10, at least 20, at least 23 or more unpaired nucleotides. Where the two substantially complementary -15- 4892-3380-3674.1
Atty. Dkt. No.: 115872-2919 strands of a dsRNA are comprised by separate RNA molecules, those molecules need not, but can be covalently connected. Where the two strands are connected covalently by means other than a hairpin loop, the connecting structure is referred to as a “linker.” The term “siRNA” is also used herein to refer to a dsRNA as described above. [0052] The term “antisense strand” or “guide strand” refers to the strand of an iRNA, e.g., a dsRNA, which includes a region that is substantially complementary to a target sequence. As used herein, the term “region of complementarity” refers to the region on the antisense strand that is substantially complementary to a sequence, for example a target sequence, as defined herein. Where the region of complementarity is not fully complementary to the target sequence, the mismatches may be in the internal or terminal regions of the molecule. Generally, the most tolerated mismatches are in the terminal regions, e.g., within 5, 4, 3, or 2 nucleotides of the 5′ and/or 3′ terminus. The term “sense strand,” or “passenger strand” as used herein, refers to the strand of an iRNA that includes a region that is substantially complementary to a region of the antisense strand as that term is defined herein. [0053] As used herein, the term “effective amount” refers to a quantity sufficient to achieve a desired therapeutic and/or prophylactic effect, e.g., an amount which results in the prevention of, or a decrease in a disease or condition described herein or one or more signs or symptoms associated with a disease or condition described herein. In the context of therapeutic or prophylactic applications, the amount of a composition administered to the subject will vary depending on the composition, the degree, type, and severity of the disease and on the characteristics of the individual, such as general health, age, sex, body weight and tolerance to drugs. The skilled artisan will be able to determine appropriate dosages depending on these and other factors. The compositions can also be administered in combination with one or more additional therapeutic compounds. As used herein, a “therapeutically effective amount” of a composition refers to composition levels in which the physiological effects of a disease or condition are ameliorated or eliminated. A therapeutically effective amount can be given in one or more administrations. -16- 4892-3380-3674.1
Atty. Dkt. No.: 115872-2919 [0054] As used herein, “expression” includes one or more of the following: transcription of the gene into precursor mRNA; splicing and other processing of the precursor mRNA to produce mature mRNA; mRNA stability; translation of the mature mRNA into protein (including codon usage and tRNA availability); and glycosylation and/or other modifications of the translation product, if required for proper expression and function. [0055] As used herein, the term “gene” means a segment of DNA that contains all the information for the regulated biosynthesis of an RNA product, including promoters, exons, introns, and other untranslated regions that control expression. [0056] “Homology” or “identity” or “similarity” refers to sequence similarity between two peptides or between two nucleic acid molecules. Homology can be determined by comparing a position in each sequence which may be aligned for purposes of comparison. When a position in the compared sequence is occupied by the same nucleobase or amino acid, then the molecules are homologous at that position. A degree of homology between sequences is a function of the number of matching or homologous positions shared by the sequences. A polynucleotide or polynucleotide region (or a polypeptide or polypeptide region) has a certain percentage (for example, at least 60%, 65%, 70%, 75%, 80%, 85%, 90%, 95%, 98% or 99%) of “sequence identity” to another sequence means that, when aligned, that percentage of bases (or amino acids) are the same in comparing the two sequences. This alignment and the percent homology or sequence identity can be determined using software programs known in the art. In some embodiments, default parameters are used for alignment. One alignment program is BLAST, using default parameters. In particular, programs are BLASTN and BLASTP, using the following default parameters: Genetic code=standard; filter=none; strand=both; cutoff=60; expect=10; Matrix=BLOSUM62; Descriptions=50 sequences; sort by ═HIGH SCORE; Databases=non-redundant, GenBank+EMBL+DDBJ+PDB+GenBank CDS translations+SwissProtein+SPupdate+PIR. Details of these programs can be found at the National Center for Biotechnology Information. Biologically equivalent polynucleotides are those having the specified percent homology and encoding a polypeptide having the same or -17- 4892-3380-3674.1
Atty. Dkt. No.: 115872-2919 similar biological activity. Two sequences are deemed “unrelated” or “non-homologous” if they share less than 40% identity, or less than 25% identity, with each other. [0057] The term “hybridize” as used herein refers to a process where two substantially complementary nucleic acid strands (at least about 65% complementary over a stretch of at least 14 to 25 nucleotides, at least about 75%, or at least about 90% complementary) anneal to each other under appropriately stringent conditions to form a duplex or heteroduplex through formation of hydrogen bonds between complementary base pairs. Nucleic acid hybridization techniques are well known in the art. See, e.g., Sambrook, et al., 1989, Molecular Cloning: A Laboratory Manual, Second Edition, Cold Spring Harbor Press, Plainview, N.Y. Hybridization and the strength of hybridization (i.e., the strength of the association between the nucleic acids) is influenced by such factors as the degree of complementarity between the nucleic acids, stringency of the conditions involved, and the thermal melting point (T
m) of the formed hybrid. Those skilled in the art understand how to estimate and adjust the stringency of hybridization conditions such that sequences having at least a desired level of complementarity will stably hybridize, while those having lower complementarity will not. For examples of hybridization conditions and parameters, see, e.g., Sambrook, et al., 1989, Molecular Cloning: A Laboratory Manual, Second Edition, Cold Spring Harbor Press, Plainview, N.Y.; Ausubel, F. M. et al.1994, Current Protocols in Molecular Biology, John Wiley & Sons, Secaucus, N.J. In some embodiments, specific hybridization occurs under stringent hybridization conditions. An oligonucleotide or polynucleotide (e.g., a probe or a primer) that is specific for a target nucleic acid will “hybridize” to the target nucleic acid under suitable conditions. [0058] As used herein, the term “iRNA,” “RNAi”, “iRNA agent,” or “RNAi agent” refers to an agent that contains RNA, and which mediates the targeted cleavage of an RNA transcript, e.g., via an RNA-induced silencing complex (RISC) pathway. In one embodiment, an iRNA as described herein effects inhibition of expression of a disease-inducing gene. Inhibition of disease-inducing gene expression may be assessed based on a reduction in the level of mRNA protein of the disease-inducing gene. The RNAi agent may be a siRNA or a miRNA. -18- 4892-3380-3674.1
Atty. Dkt. No.: 115872-2919 [0059] In another embodiment, the iRNA agent may be a “single-stranded siRNA” that is introduced into a cell or organism to inhibit a target mRNA. Single-stranded RNAi agents bind to the RISC endonuclease Argonaute 2, which then cleaves the target mRNA. The single- stranded siRNAs are generally 15-30 nucleotides and are chemically modified. The design and testing of single-stranded siRNAs are described in U.S. Pat. No.8,101,348 and in Lima et al., (2012) Cell 150: 883-894, the entire contents of each of which are hereby incorporated herein by reference. Any of the antisense nucleotide sequences described herein may be used as a single- stranded siRNA as described herein or as chemically modified by the methods described in Lima et al., (2012) Cell 150:883-894. [0060] In another aspect, the iRNA agent is a “single-stranded antisense RNA molecule”. An single-stranded antisense RNA molecule is complementary to a sequence within the target mRNA. Single-stranded antisense RNA molecules can inhibit translation in a stoichiometric manner by base pairing to the mRNA and physically obstructing the translation machinery, see Dias, N. et al., (2002) Mol Cancer Ther 1:347-355. Alternatively, the single-stranded antisense molecules inhibit a target mRNA by hydridizing to the target and cleaving the target through an RNaseH cleavage event. The single-stranded antisense RNA molecule may be about 10 to about 30 nucleotides in length and have a sequence that is complementary to a target sequence. For example, the single-stranded antisense RNA molecule may comprise a sequence that is at least about 10, 11, 12, 13, 14, 15, 16, 17, 18, 19, 20, or more contiguous nucleotides from any one of the antisense nucleotide sequences described herein. [0061] In one embodiment, an iRNA for inhibiting expression of a disease-inducing gene includes at least two sequences that are complementary to each other. The iRNA includes a sense strand having a first sequence and an antisense strand having a second sequence. The antisense strand includes a nucleotide sequence that is substantially complementary to at least part of an mRNA encoding a transcript of a disease-inducing gene, and the region of complementarity is 30 nucleotides or less, and at least 15 nucleotides in length. Generally, the iRNA is 19 to 24 nucleotides in length. In some embodiments, the iRNA is 19-21 nucleotides in length. In some embodiments, the iRNA is 19-21 nucleotides in length and is in a lipid -19- 4892-3380-3674.1
Atty. Dkt. No.: 115872-2919 formulation, e.g. a lipid nanoparticle (LNP) formulation (e.g., an LNP11 formulation). In some embodiments, the iRNA is 21-23 nucleotides in length. In some embodiments, the iRNA is 21- 23 nucleotides in length and is in the form of a conjugate, e.g., conjugated to one or more GalNAc derivatives as described herein. [0062] In some embodiments the iRNA is from about 15 to about 25 nucleotides in length, and in other embodiments the iRNA is from about 25 to about 30 nucleotides in length. An iRNA targeting a disease-inducing gene, upon contact with a cell expressing the disease-inducing gene, inhibits the expression of the disease-inducing gene by at least 10%, at least 20%, at least 25%, at least 30%, at least 35% or at least 40% or more, such as when assayed by a method as described herein. In one embodiment, the iRNA targeting the disease-inducing gene is formulated in a stable nucleic acid lipid particle (SNALP). [0063] The iRNA molecules featured herein can include naturally occurring nucleotides or can include at least one modified nucleotide, including, but not limited to a 2′-O-methyl modified nucleotide, a nucleotide having a 5′-phosphorothioate group, and a terminal nucleotide linked to a cholesteryl derivative. Alternatively, the modified nucleotide may be chosen from the group of: a 2′-deoxy-2′-fluoro modified nucleotide, a 2′-deoxy-modified nucleotide, a locked nucleotide, an abasic nucleotide, 2′-amino-modified nucleotide, 2′-alkyl-modified nucleotide, morpholino nucleotide, a phosphoramidate, and a non-natural base comprising nucleotide. [0064] As used herein, the term “nucleotide overhang” refers to at least one unpaired nucleotide that protrudes from the duplex structure of an iRNA, e.g., a dsRNA. For example, when a 3′-end of one strand of a dsRNA extends beyond the 5′-end of the other strand, or vice versa, there is a nucleotide overhang. A dsRNA can comprise an overhang of at least one nucleotide; alternatively the overhang can comprise at least two nucleotides, at least three nucleotides, at least four nucleotides, at least five nucleotides or more. A nucleotide overhang can comprise or consist of a nucleotide/nucleoside analog, including a deoxynucleotide/nucleoside. The overhang(s) may be on the sense strand, the antisense strand or any combination thereof. Furthermore, the nucleotide(s) of an overhang can be present on the 5′ end, 3′ end or both ends of either an antisense or sense strand of a dsRNA. In one embodiment, the antisense strand of a -20- 4892-3380-3674.1
Atty. Dkt. No.: 115872-2919 dsRNA has a 1-10 nucleotide overhang at the 3′ end and/or the 5′ end. In one embodiment, the sense strand of a dsRNA has a 1-10 nucleotide overhang at the 3′ end and/or the 5′ end. In another embodiment, one or more of the nucleotides in the overhang is replaced with a nucleoside thiophosphate. [0065] As used herein, “oligonucleotide” refers to a molecule that has a sequence of nucleic acid bases on a backbone comprised mainly of identical monomer units at defined intervals. The bases are arranged on the backbone in such a way that they can bind with a nucleic acid having a sequence of bases that are complementary to the bases of the oligonucleotide. The most common oligonucleotides have a backbone of sugar phosphate units. A distinction may be made between oligodeoxyribonucleotides that do not have a hydroxyl group at the 2' position and oligoribonucleotides that have a hydroxyl group at the 2' position. Oligonucleotides may also include derivatives, in which the hydrogen of the hydroxyl group is replaced with organic groups, e.g., an allyl group. One or more bases of the oligonucleotide may also be modified to include a phosphorothioate bond (e.g., one of the two oxygen atoms in the phosphate backbone which is not involved in the internucleotide bridge, is replaced by a sulfur atom) to increase resistance to nuclease degradation. The exact size of the oligonucleotide will depend on many factors, which in turn depend on the ultimate function or use of the oligonucleotide. The oligonucleotide may be generated in any manner, including, for example, chemical synthesis, DNA replication, restriction endonuclease digestion of plasmids or phage DNA, reverse transcription, PCR, or a combination thereof. The oligonucleotide may be modified e.g., by addition of a methyl group, a biotin or digoxigenin moiety, a fluorescent tag or by using radioactive nucleotides. [0066] As used herein, the term “pharmaceutically-acceptable carrier” is intended to include any and all solvents, dispersion media, coatings, antibacterial and antifungal compounds, isotonic and absorption delaying compounds, and the like, compatible with pharmaceutical administration. Pharmaceutically-acceptable carriers and their formulations are known to one skilled in the art and are described, for example, in Remington's Pharmaceutical Sciences (20
th edition, ed. A. Gennaro, 2000, Lippincott, Williams & Wilkins, Philadelphia, Pa.). -21- 4892-3380-3674.1
Atty. Dkt. No.: 115872-2919 [0067] As used herein, the term “polynucleotide” or “nucleic acid” means any RNA or DNA, which may be unmodified or modified RNA or DNA. Polynucleotides include, without limitation, single- and double-stranded DNA, DNA that is a mixture of single- and double- stranded regions, single- and double-stranded RNA, RNA that is mixture of single- and double- stranded regions, and hybrid molecules comprising DNA and RNA that may be single-stranded or, more typically, double-stranded or a mixture of single- and double-stranded regions. In addition, polynucleotide refers to triple-stranded regions comprising RNA or DNA or both RNA and DNA. The term polynucleotide also includes DNAs or RNAs containing one or more modified bases and DNAs or RNAs with backbones modified for stability or for other reasons. [0068] The term “RNA molecule” or “ribonucleic acid molecule” encompasses not only RNA molecules as expressed or found in nature, but also analogs and derivatives of RNA comprising one or more ribonucleotide/ribonucleoside analogs or derivatives as described herein or as known in the art. Strictly speaking, a “ribonucleoside” includes a nucleoside base and a ribose sugar, and a “ribonucleotide” is a ribonucleoside with one, two or three phosphate moieties. However, the terms “ribonucleoside” and “ribonucleotide” can be considered to be equivalent as used herein. The RNA can be modified in the nucleobase structure or in the ribose- phosphate backbone structure, e.g., as described herein below. However, the molecules comprising ribonucleoside analogs or derivatives must retain the ability to form a duplex. As non-limiting examples, an RNA molecule can also include at least one modified ribonucleoside including but not limited to a 2′-O-methyl modified nucleostide, a nucleoside comprising a 5′ phosphorothioate group, a terminal nucleoside linked to a cholesteryl derivative or dodecanoic acid bisdecylamide group, a locked nucleoside, an abasic nucleoside, a 2′-deoxy-2′-fluoro modified nucleoside, a 2′-amino-modified nucleoside, 2′-alkyl-modified nucleoside, morpholino nucleoside, a phosphoramidate or a non-natural base comprising nucleoside, or any combination thereof. Alternatively, an RNA molecule can comprise at least two modified ribonucleosides, at least 3, at least 4, at least 5, at least 6, at least 7, at least 8, at least 9, at least 10, at least 15, at least 20 or more, up to the entire length of the dsRNA molecule. The modifications need not be the same for each of such a plurality of modified ribonucleosides in an RNA molecule. In one embodiment, modified RNAs contemplated for use in methods and compositions described -22- 4892-3380-3674.1
Atty. Dkt. No.: 115872-2919 herein are peptide nucleic acids (PNAs) that have the ability to form the required duplex structure and that permit or mediate the specific degradation of a target RNA, e.g., via a RISC pathway. [0069] In one aspect, a modified ribonucleoside includes a deoxyribonucleoside. In such an instance, an iRNA agent can comprise one or more deoxynucleosides, including, for example, a deoxynucleoside overhang(s), or one or more deoxynucleosides within the double stranded portion of a dsRNA. However, it is self evident that under no circumstances is a double stranded DNA molecule encompassed by the term “iRNA.” [0070] In one aspect, an RNA interference agent includes a single stranded RNA that interacts with a target RNA sequence to direct the cleavage of the target RNA. Without wishing to be bound by theory, long double stranded RNA introduced into cells is broken down into siRNA by a Type III endonuclease known as Dicer (Sharp et al., Genes Dev.2001, 15:485). Dicer, a ribonuclease-III-like enzyme, processes the dsRNA into 19-23 base pair short interfering RNAs with characteristic two base 3′ overhangs (Bernstein, et al., (2001) Nature 409:363). The siRNAs are then incorporated into an RNA-induced silencing complex (RISC) where one or more helicases unwind the siRNA duplex, enabling the complementary antisense strand to guide target recognition (Nykanen, et al., (2001) Cell 107:309). Upon binding to the appropriate target mRNA, one or more endonucleases within the RISC cleaves the target to induce silencing (Elbashir, et al., (2001) Genes Dev.15:188). Thus, in one aspect the present disclosure relates to a single stranded RNA that promotes the formation of a RISC complex to effect silencing of the target gene. [0071] As used herein, the term “sample” refers to clinical samples obtained from a subject. Biological samples may include tissues, cells, protein or membrane extracts of cells, mucus, sputum, bone marrow, bronchial alveolar lavage (BAL), bronchial wash (BW), and biological fluids (e.g., ascites fluid or cerebrospinal fluid (CSF)) isolated from a subject, as well as tissues, cells and fluids (blood, plasma, saliva, urine, serum etc.) present within a subject. [0072] As used herein, the term “separate” therapeutic use refers to an administration of at least two active ingredients at the same time or at substantially the same time by different routes. -23- 4892-3380-3674.1
Atty. Dkt. No.: 115872-2919 [0073] As used herein, the term “sequential” therapeutic use refers to administration of at least two active ingredients at different times, the administration route being identical or different. More particularly, sequential use refers to the whole administration of one of the active ingredients before administration of the other or others commences. It is thus possible to administer one of the active ingredients over several minutes, hours, or days before administering the other active ingredient or ingredients. There is no simultaneous treatment in this case. [0074] As used herein, the term “simultaneous” therapeutic use refers to the administration of at least two active ingredients by the same route and at the same time or at substantially the same time. [0075] As used herein, the term “SNALP” refers to a stable nucleic acid-lipid particle. A SNALP represents a vesicle of lipids coating a reduced aqueous interior comprising a nucleic acid such as an iRNA or a plasmid from which an iRNA is transcribed. SNALPs are described, e.g., in U.S. Patent Application Publication Nos.20060240093, 20070135372, and in International Application No. WO 2009082817. These applications are incorporated herein by reference in their entirety. [0076] The term “specific” as used herein in reference to an oligonucleotide means that the nucleotide sequence of the oligonucleotide has at least 12 bases of sequence identity with a portion of a target nucleic acid when the oligonucleotide and the target nucleic acid are aligned. An oligonucleotide that is specific for a target nucleic acid is one that, under the stringent hybridization or washing conditions, is capable of hybridizing to the target nucleic acid of interest and not substantially hybridizing to nucleic acids which are not of interest. Higher levels of sequence identity are desirable and include at least 75%, at least 80%, at least 85%, at least 90%, at least 95% or at least 98% sequence identity. [0077] The term “stringent hybridization conditions” as used herein refers to hybridization conditions at least as stringent as the following: hybridization in 50% formamide, 5xSSC, 50 mM NaH
2PO
4, pH 6.8, 0.5% SDS, 0.1 mg/mL sonicated salmon sperm DNA, and 5x Denhart's solution at 42
o C overnight; washing with 2x SSC, 0.1% SDS at 45
o C; and washing with 0.2x -24- 4892-3380-3674.1
Atty. Dkt. No.: 115872-2919 SSC, 0.1% SDS at 45
o C. In another example, stringent hybridization conditions should not allow for hybridization of two nucleic acids which differ over a stretch of 20 contiguous nucleotides by more than two bases. [0078] As used herein, the terms “subject,” “individual,” or “patient” are used interchangeably and refer to an individual organism, a vertebrate, a mammal, or a human. In certain embodiments, the individual, patient or subject is a human. [0079] As used herein, the terms “target sequence” and “target nucleic acid sequence” refer to a specific nucleic acid sequence to be modulated (e.g., inhibited or downregulated). In some embodiments, the target sequence refers to a contiguous portion of the nucleotide sequence of an mRNA molecule formed during the transcription of a target gene, including mRNA that is a product of RNA processing of a primary transcription product. The target portion of the sequence will be at least long enough to serve as a substrate for iRNA-directed cleavage at or near that portion. For example, the target sequence will generally be from 9-36 nucleotides in length, e.g., 15-30 nucleotides in length, including all sub-ranges therebetween. As non-limiting examples, the target sequence can be from 15-30 nucleotides, 15-26 nucleotides, 15-23 nucleotides, 15-22 nucleotides, 15-21 nucleotides, 15-20 nucleotides, 15-19 nucleotides, 15-18 nucleotides, 15-17 nucleotides, 18-30 nucleotides, 18-26 nucleotides, 18-23 nucleotides, 18-22 nucleotides, 18-21 nucleotides, 18-20 nucleotides, 19-30 nucleotides, 19-26 nucleotides, 19-23 nucleotides, 19-22 nucleotides, 19-21 nucleotides, 19-20 nucleotides, 20-30 nucleotides, 20-26 nucleotides, 20-25 nucleotides, 20-24 nucleotides, 20-23 nucleotides, 20-22 nucleotides, 20-21 nucleotides, 21-30 nucleotides, 21-26 nucleotides, 21-25 nucleotides, 21-24 nucleotides, 21-23 nucleotides, or 21-22 nucleotides. [0080] “Treating”, “treat”, or “treatment” as used herein covers the treatment of a disease or disorder described herein, in a subject, such as a human, and includes: (i) inhibiting a disease or disorder, i.e., arresting its development; (ii) relieving a disease or disorder, i.e., causing regression of the disorder; (iii) slowing progression of the disorder; and/or (iv) inhibiting, relieving, or slowing progression of one or more symptoms of the disease or disorder. In some -25- 4892-3380-3674.1
Atty. Dkt. No.: 115872-2919 embodiments, treatment means that the symptoms associated with the disease are, e.g., alleviated, reduced, cured, or placed in a state of remission. [0081] It is also to be appreciated that the various modes of treatment or prevention of medical diseases and conditions as described are intended to mean “substantial,” which includes total but also less than total treatment or prevention, and wherein some biologically or medically relevant result is achieved. The treatment may be a continuous prolonged treatment for a chronic disease or a single, or few time administrations for the treatment of an acute condition. RNAi Agents [0082] A dsRNA includes two RNA strands that are sufficiently complementary to hybridize to form a duplex structure under conditions in which the dsRNA will be used. One strand of a dsRNA (the antisense strand) includes a region of complementarity that is substantially complementary, and generally fully complementary, to a target sequence, derived from the sequence of an mRNA formed during the expression of a disease-inducing gene. The other strand (the sense strand) includes a region that is complementary to the antisense strand, such that the two strands hybridize and form a duplex structure when combined under suitable conditions. Generally, the duplex structure is between 15 and 30 inclusive, more generally between 18 and 25 inclusive, yet more generally between 19 and 24 inclusive, and most generally between 19 and 21 base pairs in length, inclusive. Similarly, the region of complementarity to the target sequence is between 15 and 30 inclusive, more generally between 18 and 25 inclusive, yet more generally between 19 and 24 inclusive, and most generally between 19 and 21 nucleotides in length, inclusive. In some embodiments, the dsRNA is between 15 and 20 nucleotides in length, inclusive, and in other embodiments, the dsRNA is between 25 and 30 nucleotides in length, inclusive. As the ordinarily skilled person will recognize, the targeted region of an RNA targeted for cleavage will most often be part of a larger RNA molecule, often an mRNA molecule. Where relevant, a “part” of an mRNA target is a contiguous sequence of an mRNA target of sufficient length to be a substrate for RNAi-directed cleavage (i.e., cleavage through a RISC pathway). dsRNAs having duplexes as short as 9 base -26- 4892-3380-3674.1
Atty. Dkt. No.: 115872-2919 pairs can, under some circumstances, mediate RNAi-directed RNA cleavage. Most often a target will be at least 15 nucleotides in length, e.g., 15-30 nucleotides in length. [0083] One of skill in the art will also recognize that the duplex region is a primary functional portion of a dsRNA, e.g., a duplex region of 9 to 36, e.g., 15-30 base pairs. Thus, in one embodiment, to the extent that it becomes processed to a functional duplex of e.g., 15-30 base pairs that targets a desired RNA for cleavage, an RNA molecule or complex of RNA molecules having a duplex region greater than 30 base pairs is a dsRNA. Thus, an ordinarily skilled artisan will recognize that in one embodiment, then, an miRNA is a dsRNA. In another embodiment, a dsRNA is not a naturally occurring miRNA. In another embodiment, an iRNA agent useful to target disease-inducing gene expression is not generated in the target cell by cleavage of a larger dsRNA. [0084] In these aspects, one of the two sequences is complementary to the other of the two sequences, with one of the sequences being substantially complementary to a sequence of an mRNA generated by the expression of a disease-inducing gene. As described elsewhere herein and as known in the art, the complementary sequences of a dsRNA can also be contained as self- complementary regions of a single nucleic acid molecule, as opposed to being on separate oligonucleotides. [0085] While a target sequence is generally 15-30 nucleotides in length, there is wide variation in the suitability of particular sequences in this range for directing cleavage of any given target RNA. Various software packages and the guidelines set out herein provide guidance for the identification of optimal target sequences for any given gene target, but an empirical approach can also be taken in which a “window” or “mask” of a given size (as a non-limiting example, 21 nucleotides) is literally or figuratively (including, e.g., in silico) placed on the target RNA sequence to identify sequences in the size range that may serve as target sequences. By moving the sequence “window” progressively one nucleotide upstream or downstream of an initial target sequence location, the next potential target sequence can be identified, until the complete set of possible sequences is identified for any given target size selected. This process, coupled with systematic synthesis and testing of the identified sequences (using assays as -27- 4892-3380-3674.1
Atty. Dkt. No.: 115872-2919 described herein or as known in the art) to identify those sequences that perform optimally can identify those RNA sequences that, when targeted with an iRNA agent, mediate the best inhibition of target gene expression. [0086] Further still, such optimized sequences can be adjusted by, e.g., the introduction of modified nucleotides as described herein or as known in the art, addition or changes in overhang, or other modifications as known in the art and/or discussed herein to further optimize the molecule (e.g., increasing serum stability or circulating half-life, increasing thermal stability, enhancing transmembrane delivery, targeting to a particular location or cell type, increasing interaction with silencing pathway enzymes, increasing release from endosomes, etc.) as an expression inhibitor. [0087] An iRNA as described herein can contain one or more mismatches to the target sequence. In one embodiment, an iRNA as described herein contains no more than 3 mismatches. If the antisense strand of the iRNA contains mismatches to a target sequence, it is preferable that the area of mismatch not be located in the center of the region of complementarity. If the antisense strand of the iRNA contains mismatches to the target sequence, it is preferable that the mismatch be restricted to be within the last 5 nucleotides from either the 5′ or 3′ end of the region of complementarity. For example, for a 23 nucleotide iRNA agent RNA strand which is complementary to a region of a disease-inducing gene, the RNA strand generally does not contain any mismatch within the central 13 nucleotides. The methods described herein or methods known in the art can be used to determine whether an iRNA containing a mismatch to a target sequence is effective in inhibiting the expression of a disease- inducing gene. Consideration of the efficacy of iRNAs with mismatches in inhibiting expression of a disease-inducing gene is important, especially if the particular region of complementarity in a disease-inducing gene is known to have polymorphic sequence variation within the population. [0088] In one embodiment, at least one end of a dsRNA has a single-stranded nucleotide overhang of 1 to 4, generally 1 or 2 nucleotides. dsRNAs having at least one nucleotide overhang have unexpectedly superior inhibitory properties relative to their blunt-ended counterparts. In yet another embodiment, the RNA of an iRNA, e.g., a dsRNA, is chemically -28- 4892-3380-3674.1
Atty. Dkt. No.: 115872-2919 modified to enhance stability or other beneficial characteristics. The nucleic acids featured in the present technology may be synthesized and/or modified by methods well established in the art, such as those described in “Current protocols in nucleic acid chemistry,” Beaucage, S. L. et al. (Edrs.), John Wiley & Sons, Inc., New York, N.Y., USA, which is hereby incorporated herein by reference. Modifications include, for example, (a) end modifications, e.g., 5′ end modifications (phosphorylation, conjugation, inverted linkages, etc.) 3′ end modifications (conjugation, DNA nucleotides, inverted linkages, etc.), (b) base modifications, e.g., replacement with stabilizing bases, destabilizing bases, or bases that base pair with an expanded repertoire of partners, removal of bases (abasic nucleotides), or conjugated bases, (c) sugar modifications (e.g., at the 2′ position or 4′ position) or replacement of the sugar, as well as (d) backbone modifications, including modification or replacement of the phosphodiester linkages. Specific examples of RNA compounds useful in the present technology include, but are not limited to RNAs containing modified backbones or no natural internucleoside linkages. RNAs having modified backbones include, among others, those that do not have a phosphorus atom in the backbone. For the purposes of this specification, and as sometimes referenced in the art, modified RNAs that do not have a phosphorus atom in their internucleoside backbone can also be considered to be oligonucleosides. In particular embodiments, the modified RNA will have a phosphorus atom in its internucleoside backbone. [0089] Modified RNA backbones include, for example, phosphorothioates, chiral phosphorothioates, phosphorodithioates, phosphotriesters, aminoalkylphosphotriesters, methyl and other alkyl phosphonates including 3′-alkylene phosphonates and chiral phosphonates, phosphinates, phosphoramidates including 3′-amino phosphoramidate and aminoalkylphosphoramidates, thionophosphoramidates, thionoalkylphosphonates, thionoalkylphosphotriesters, and boranophosphates having normal 3′-5′ linkages, 2′-5′ linked analogs of these, and those) having inverted polarity wherein the adjacent pairs of nucleoside units are linked 3′-5′ to 5′-3′ or 2′-5′ to 5′-2′. Various salts, mixed salts and free acid forms are also included. -29- 4892-3380-3674.1
Atty. Dkt. No.: 115872-2919 [0090] Representative U.S. patents that teach the preparation of the above phosphorus- containing linkages include, but are not limited to, U.S. Pat. Nos.3,687,808; 4,469,863; 4,476,301; 5,023,243; 5,177,195; 5,188,897; 5,264,423; 5,276,019; 5,278,302; 5,286,717; 5,321,131; 5,399,676; 5,405,939; 5,453,496; 5,455,233; 5,466,677; 5,476,925; 5,519,126; 5,536,821; 5,541,316; 5,550,111; 5,563,253; 5,571,799; 5,587,361; 5,625,050; 6,028,188; 6,124,445; 6,160,109; 6,169,170; 6,172,209; 6,239,265; 6,277,603; 6,326,199; 6,346,614; 6,444,423; 6,531,590; 6,534,639; 6,608,035; 6,683,167; 6,858,715; 6,867,294; 6,878,805; 7,015,315; 7,041,816; 7,273,933; 7,321,029; and U.S. Pat. No. RE39464, each of which is herein incorporated by reference. [0091] Modified RNA backbones that do not include a phosphorus atom therein have backbones that are formed by short chain alkyl or cycloalkyl internucleoside linkages, mixed heteroatoms and alkyl or cycloalkyl internucleoside linkages, or one or more short chain heteroatomic or heterocyclic internucleoside linkages. These include those having morpholino linkages (formed in part from the sugar portion of a nucleoside); siloxane backbones; sulfide, sulfoxide and sulfone backbones; formacetyl and thioformacetyl backbones; methylene formacetyl and thioformacetyl backbones; alkene containing backbones; sulfamate backbones; methyleneimino and methylenehydrazino backbones; sulfonate and sulfonamide backbones; amide backbones; and others having mixed N, O, S and CH
2 component parts. [0092] Representative U.S. patents that teach the preparation of the above oligonucleosides include, but are not limited to, U.S. Pat. Nos.5,034,506; 5,166,315; 5,185,444; 5,214,134; 5,216,141; 5,235,033; 5,64,562; 5,264,564; 5,405,938; 5,434,257; 5,466,677; 5,470,967; 5,489,677; 5,541,307; 5,561,225; 5,596,086; 5,602,240; 5,608,046; 5,610,289; 5,618,704; 5,623,070; 5,663,312; 5,633,360; 5,677,437; and, 5,677,439, each of which is herein incorporated by reference. [0093] In other RNA mimetics suitable or contemplated for use in iRNAs, both the sugar and the internucleoside linkage, i.e., the backbone, of the nucleotide units are replaced with novel groups. The base units are maintained for hybridization with an appropriate nucleic acid target compound. One such oligomeric compound, an RNA mimetic that has been shown to have -30- 4892-3380-3674.1
Atty. Dkt. No.: 115872-2919 excellent hybridization properties, is referred to as a peptide nucleic acid (PNA). In PNA compounds, the sugar backbone of an RNA is replaced with an amide containing backbone, in particular an aminoethylglycine backbone. The nucleobases are retained and are bound directly or indirectly to aza nitrogen atoms of the amide portion of the backbone. Representative U.S. patents that teach the preparation of PNA compounds include, but are not limited to, U.S. Pat. Nos.5,539,082; 5,714,331; and 5,719,262, each of which is herein incorporated by reference. Further teaching of PNA compounds can be found, for example, in Nielsen et al., Science, 1991, 254, 1497-1500. [0094] Some embodiments featured in the present technology include RNAs with phosphorothioate backbones and oligonucleosides with heteroatom backbones, and in particular —CH
2—NH—CH
2—, —CH
2—N(CH
3)—O—CH
2—[known as a methylene (methylimino) or MMI backbone], —CH
2—O—N(CH
3)—CH
2—, —CH
2—N(CH
3)—N(CH
3)—CH
2— and — N(CH
3)—CH
2—CH
2—[wherein the native phosphodiester backbone is represented as —O— P—O—CH
2—] of the above-referenced U.S. Pat. No.5,489,677, and the amide backbones of the above-referenced U.S. Pat. No.5,602,240. In some embodiments, the RNAs featured herein have morpholino backbone structures of the above-referenced U.S. Pat. No.5,034,506. [0095] Modified RNAs may also contain one or more substituted sugar moieties. The iRNAs, e.g., dsRNAs, featured herein can include one of the following at the 2′ position: OH; F; O-, S-, or N-alkyl; O-, S-, or N-alkenyl; O-, S- or N-alkynyl; or O-alkyl-O-alkyl, wherein the alkyl, alkenyl and alkynyl may be substituted or unsubstituted C
1 to C
10 alkyl or C
2 to C
10 alkenyl and alkynyl. Exemplary suitable modifications include O[(CH
2)
nO]
mCH
3, O(CH
2).
nOCH
3, O(CH
2)
nNH
2, O(CH
2)
nCH
3, O(CH
2)
nONH
2, and O(CH
2)
nON[(CH
2)
nCH
3)]
2, where n and m are from 1 to about 10. In other embodiments, dsRNAs include one of the following at the 2′ position: C
1 to C
10 lower alkyl, substituted lower alkyl, alkaryl, aralkyl, O-alkaryl or O-aralkyl, SH, SCH
3, OCN, Cl, Br, CN, CF
3, OCF
3, SOCH
3, SO
2CH
3, ONO
2, NO
2, N
3, NH
2, heterocycloalkyl, heterocycloalkaryl, aminoalkylamino, polyalkylamino, substituted silyl, an RNA cleaving group, a reporter group, an intercalator, a group for improving the pharmacokinetic properties of an iRNA, or a group for improving the pharmacodynamic -31- 4892-3380-3674.1
Atty. Dkt. No.: 115872-2919 properties of an iRNA, and other substituents having similar properties. In some embodiments, the modification includes a 2′-methoxyethoxy (2′-O—CH
2CH
2OCH
3, also known as 2′-O-(2- methoxyethyl) or 2′-MOE) (Martin et al., Helv. Chim. Acta, 1995, 78:486-504) i.e., an alkoxy- alkoxy group. Another exemplary modification is 2′-dimethylaminooxyethoxy, i.e., a O(CH
2)
2ON(CH
3)
2 group, also known as 2′-DMAOE, as described in examples herein below, and 2′-dimethylaminoethoxyethoxy (also known in the art as 2′-O-dimethylaminoethoxyethyl or 2′-DMAEOE), i.e., 2′-O—CH
2—O-—CH
2—N(CH
2)
2, also described in examples herein below. [0096] Other modifications include 2′-methoxy (2′-OCH
3), 2′-aminopropoxy (2′- OCH
2CH
2CH
2NH
2) and 2′-fluoro (2′-F). Similar modifications may also be made at other positions on the RNA of an iRNA, particularly the 3′ position of the sugar on the 3′ terminal nucleotide or in 2′-5′ linked dsRNAs and the 5′ position of 5′ terminal nucleotide. iRNAs may also have sugar mimetics such as cyclobutyl moieties in place of the pentofuranosyl sugar. Representative U.S. patents that teach the preparation of such modified sugar structures include, but are not limited to, U.S. Pat. Nos.4,981,957; 5,118,800; 5,319,080; 5,359,044; 5,393,878; 5,446,137; 5,466,786; 5,514,785; 5,519,134; 5,567,811; 5,576,427; 5,591,722; 5,597,909; 5,610,300; 5,627,053; 5,639,873; 5,646,265; 5,658,873; 5,670,633; and 5,700,920, certain of which are commonly owned with the instant application, and each of which is herein incorporated by reference. [0097] An iRNA may also include nucleobase (often referred to in the art simply as “base”) modifications or substitutions. As used herein, “unmodified” or “natural” nucleobases include the purine bases adenine (A) and guanine (G), and the pyrimidine bases thymine (T), cytosine (C) and uracil (U). Modified nucleobases include other synthetic and natural nucleobases such as 5-methylcytosine (5-me-C), 5-hydroxymethyl cytosine, xanthine, hypoxanthine, 2- aminoadenine, 6-methyl and other alkyl derivatives of adenine and guanine, 2-propyl and other alkyl derivatives of adenine and guanine, 2-thiouracil, 2-thiothymine and 2-thiocytosine, 5- halouracil and cytosine, 5-propynyl uracil and cytosine, 6-azo uracil, cytosine and thymine, 5- uracil (pseudouracil), 4-thiouracil, 8-halo, 8-amino, 8-thiol, 8-thioalkyl, 8-hydroxyl anal other 8- substituted adenines and guanines, 5-halo, particularly 5-bromo, 5-trifluoromethyl and other 5- -32- 4892-3380-3674.1
Atty. Dkt. No.: 115872-2919 substituted uracils and cytosines, 7-methylguanine and 7-methyladenine, 8-azaguanine and 8- azaadenine, 7-deazaguanine and 7-daazaadenine and 3-deazaguanine and 3-deazaadenine. Further nucleobases include those disclosed in U.S. Pat. No.3,687,808, those disclosed in Modified Nucleosides in Biochemistry, Biotechnology and Medicine, Herdewijn, P. ed. Wiley- VCH, 2008; those disclosed in The Concise Encyclopedia Of Polymer Science And Engineering, pages 858-859, Kroschwitz, J. L, ed. John Wiley & Sons, 1990, these disclosed by Englisch et al., Angewandte Chemie, International Edition, 1991, 30, 613, and those disclosed by Sanghvi, Y S., Chapter 15, dsRNA Research and Applications, pages 289-302, Crooke, S. T. and Lebleu, B., Ed., CRC Press, 1993. Certain of these nucleobases are particularly useful for increasing the binding affinity of the oligomeric compounds featured in the present technology. These include 5-substituted pyrimidines, 6-azapyrimidines and N-2, N-6 and 0-6 substituted purines, including 2-aminopropyladenine, 5-propynyluracil and 5-propynylcytosine.5-methylcytosine substitutions have been shown to increase nucleic acid duplex stability by 0.6-1.2° C. (Sanghvi, Y. S., Crooke, S. T. and Lebleu, B., Eds., dsRNA Research and Applications, CRC Press, Boca Raton, 1993, pp.276-278) and are exemplary base substitutions, even more particularly when combined with 2′-O-methoxyethyl sugar modifications. [0098] Representative U.S. patents that teach the preparation of certain of the above noted modified nucleobases as well as other modified nucleobases include, but are not limited to, the above noted U.S. Pat. No.3,687,808, as well as U.S. Pat. Nos.4,845,205; 5,130,30; 5,134,066; 5,175,273; 5,367,066; 5,432,272; 5,457,187; 5,459,255; 5,484,908; 5,502,177; 5,525,711; 5,552,540; 5,587,469; 5,594,121, 5,596,091; 5,614,617; 5,681,941; 6,015,886; 6,147,200; 6,166,197; 6,222,025; 6,235,887; 6,380,368; 6,528,640; 6,639,062; 6,617,438; 7,045,610; 7,427,672; and 7,495,088, each of which is herein incorporated by reference, and U.S. Pat. No. 5,750,692, also herein incorporated by reference. [0099] The RNA of an iRNA can also be modified to include one or more locked nucleic acids (LNA). A locked nucleic acid is a nucleotide having a modified ribose moiety in which the ribose moiety comprises an extra bridge connecting the 2′ and 4′ carbons. This structure effectively “locks” the ribose in the 3′-endo structural conformation. The addition of locked -33- 4892-3380-3674.1
Atty. Dkt. No.: 115872-2919 nucleic acids to siRNAs has been shown to increase siRNA stability in serum, and to reduce off- target effects (Elmen, J. et al., (2005) Nucleic Acids Research 33(1):439-447; Mook, O R. et al., (2007) Mol Canc Ther 6(3):833-843; Grunweller, A. et al., (2003) Nucleic Acids Research 31(12):3185-3193). [00100] Representative U.S. Patents that teach the preparation of locked nucleic acid nucleotides include, but are not limited to, the following: U.S. Pat. Nos.6,268,490; 6,670,461; 6,794,499; 6,998,484; 7,053,207; 7,084,125; and 7,399,845, each of which is herein incorporated by reference in its entirety. [00101] Potentially stabilizing modifications to the ends of RNA molecules can include N- (acetylaminocaproyl)-4-hydroxyprolinol (Hyp-C6-NHAc), N-(caproyl-4-hydroxyprolinol (Hyp- C6), N-(acetyl-4-hydroxyprolinol (Hyp-NHAc), thymidine-2′-O-deoxythymidine (ether), N- (aminocaproyl)-4-hydroxyprolinol (Hyp-C6-amino), 2-docosanoyl-uridine-3″-phosphate, inverted base dT(idT) and others. Disclosure of this modification can be found in PCT Publication No. WO 2011/005861. [00102] iRNA Conjugates [00103] The iRNA agents disclosed herein can be in the form of conjugates. The conjugate may be attached at any suitable location in the iRNA molecule, e.g., at the 3′ end or the 5′ end of the sense or the antisense strand. The conjugates are optionally attached via a linker. [00104] In some embodiments, an iRNA agent described herein is chemically linked to one or more ligands, moieties or conjugates, which may confer functionality, e.g., by affecting (e.g., enhancing) the activity, cellular distribution or cellular uptake of the iRNA. Such moieties include but are not limited to lipid moieties such as a cholesterol moiety (Letsinger et al., Proc. Natl. Acid. Sci. USA, 1989, 86: 6553-6556), cholic acid (Manoharan et al., Biorg. Med. Chem. Let., 1994, 4:1053-1060), a thioether, e.g., beryl-5-tritylthiol (Manoharan et al., Ann. N.Y. Acad. Sci., 1992, 660:306-309; Manoharan et al., Biorg. Med. Chem. Let., 1993, 3:2765-2770), a thiocholesterol (Oberhauser et al., Nucl. Acids Res., 1992, 20:533-538), an aliphatic chain, e.g., dodecandiol or undecyl residues (Saison-Behmoaras et al., EMBO J, 1991, 10:1111-1118; Kabanov et al., FEBS Lett., 1990, 259:327-330; Svinarchuk et al., Biochimie, 1993, 75:49-54), a -34- 4892-3380-3674.1
Atty. Dkt. No.: 115872-2919 phospholipid, e.g., di-hexadecyl-rac-glycerol or triethyl-ammonium 1,2-di-O-hexadecyl-rac- glycero-3-phosphonate (Manoharan et al., Tetrahedron Lett., 1995, 36:3651-3654; Shea et al., Nucl. Acids Res., 1990, 18:3777-3783), a polyamine or a polyethylene glycol chain (Manoharan et al., Nucleosides & Nucleotides, 1995, 14:969-973), or adamantane acetic acid (Manoharan et al., Tetrahedron Lett., 1995, 36:3651-3654), a palmityl moiety (Mishra et al., Biochim. Biophys. Acta, 1995, 1264:229-237), or an octadecylamine or hexylamino-carbonyloxycholesterol moiety (Crooke et al., J. Pharmacol. Exp. Ther., 1996, 277:923-937). [00105] In one embodiment, a ligand alters the distribution, targeting or lifetime of an iRNA agent into which it is incorporated. In some embodiments, a ligand provides an enhanced affinity for a selected target, e.g, molecule, cell or cell type, compartment, e.g., a cellular or organ compartment, tissue, organ or region of the body, as, e.g., compared to a species absent such a ligand. Typical ligands will not take part in duplex pairing in a duplexed nucleic acid. [00106] Ligands can include a naturally occurring substance, such as a protein (e.g., human serum albumin (HSA), low-density lipoprotein (LDL), or globulin); carbohydrate (e.g., a dextran, pullulan, chitin, chitosan, inulin, cyclodextrin or hyaluronic acid); or a lipid. The ligand may also be a recombinant or synthetic molecule, such as a synthetic polymer, e.g., a synthetic polyamino acid. Examples of polyamino acids include polyamino acid is a polylysine (PLL), poly L-aspartic acid, poly L-glutamic acid, styrene-maleic acid anhydride copolymer, poly(L- lactide-co-glycolied) copolymer, divinyl ether-maleic anhydride copolymer, N-(2- hydroxypropyl)methacrylamide copolymer (HMPA), polyethylene glycol (PEG), polyvinyl alcohol (PVA), polyurethane, poly(2-ethylacryllic acid), N-isopropylacrylamide polymers, or polyphosphazine. Examples of polyamines include: polyethylenimine, polylysine (PLL), spermine, spermidine, polyamine, pseudopeptide-polyamine, peptidomimetic polyamine, dendrimer polyamine, arginine, amidine, protamine, cationic lipid, cationic porphyrin, quaternary salt of a polyamine, or an α helical peptide. [00107] Ligands can also include targeting groups, e.g., a cell or tissue targeting agent, e.g., a lectin, glycoprotein, lipid or protein, e.g., an antibody, that binds to a specified cell type such as a kidney cell. A targeting group can be a thyrotropin, melanotropin, lectin, glycoprotein, -35- 4892-3380-3674.1
Atty. Dkt. No.: 115872-2919 surfactant protein A, Mucin carbohydrate, multivalent lactose, multivalent galactose, N-acetyl- galactosamine, N-acetyl-glucosamine multivalent mannose, multivalent fucose, glycosylated polyaminoacids, multivalent galactose, transferrin, bisphosphonate, polyglutamate, polyaspartate, a lipid, cholesterol, a steroid, bile acid, folate, vitamin B12, biotin, or an RGD peptide or RGD peptide mimetic. [00108] In some embodiments, an iRNA oligonucleotide further comprises a carbohydrate. The carbohydrate conjugated iRNA are advantageous for the in vivo delivery of nucleic acids, as well as compositions suitable for in vivo therapeutic use, as described herein. [00109] As used herein, “carbohydrate” refers to a compound which is either a carbohydrate per se made up of one or more monosaccharide units having at least 6 carbon atoms (which can be linear, branched or cyclic) with an oxygen, nitrogen or sulfur atom bonded to each carbon atom; or a compound having as a part thereof a carbohydrate moiety made up of one or more monosaccharide units each having at least six carbon atoms (which can be linear, branched or cyclic), with an oxygen, nitrogen or sulfur atom bonded to each carbon atom. Representative carbohydrates include the sugars (mono-, di-, tri- and oligosaccharides containing from about 4, 5, 6, 7, 8, or 9 monosaccharide units), and polysaccharides such as starches, glycogen, cellulose and polysaccharide gums. Specific monosaccharides include C5 and above (e.g., C5, C6, C7, or C8) sugars; di- and trisaccharides include sugars having two or three monosaccharide units (e.g., C5, C6, C7, or C8). In one embodiment, a carbohydrate conjugate comprises a monosaccharide. In one embodiment, the monosaccharide is an N-acetylgalactosamine (GalNAc). GalNAc conjugates are described, for example, in U.S. Pat. No.8,106,022, the entire content of which is hereby incorporated herein by reference. In some embodiments, the GalNAc conjugate serves as a ligand that targets the iRNA to particular cells. In some embodiments, the GalNAc conjugate targets the iRNA to liver cells, e.g., by serving as a ligand for the asialoglycoprotein receptor of liver cells (e.g., hepatocytes). In some embodiments, the carbohydrate conjugate comprises one or more GalNAc derivatives. The GalNAc derivatives may be attached via a linker, e.g., a bivalent or trivalent branched linker. In some embodiments the GalNAc conjugate is conjugated to the 3′ end of the sense strand. In some embodiments, the GalNAc conjugate is conjugated to -36- 4892-3380-3674.1
Atty. Dkt. No.: 115872-2919 the iRNA agent (e.g., to the 3′ end of the sense strand) via a linker, e.g., a linker as described herein. [00110] In some embodiments, the ligand is a GalNAc ligand that comprises one or more N- acetylgalactosamine (GalNAc) derivatives. [00111] Other examples of ligands include dyes, intercalating agents (e.g., acridines), cross- linkers (e.g., psoralene, mitomycin C), porphyrins (TPPC4, texaphyrin, Sapphyrin), polycyclic aromatic hydrocarbons (e.g., phenazine, dihydrophenazine), artificial endonucleases (e.g., EDTA), lipophilic molecules, e.g, cholesterol, cholic acid, adamantane acetic acid, 1-pyrene butyric acid, dihydrotestosterone, 1,3-Bis-O(hexadecyl)glycerol, geranyloxyhexyl group, hexadecylglycerol, borneol, menthol, 1,3-propanediol, heptadecyl group, palmitic acid, myristic acid, O3-(oleoyl)lithocholic acid, O3-(oleoyl)cholenic acid, dimethoxytrityl, or phenoxazine) and peptide conjugates (e.g., antennapedia peptide, Tat peptide), alkylating agents, phosphate, amino, mercapto, PEG (e.g., PEG-40K), MPEG, [MPEG]
2, polyamino, alkyl, substituted alkyl, radiolabeled markers, enzymes, haptens (e.g., biotin), transport/absorption facilitators (e.g., aspirin, vitamin E, folic acid), synthetic ribonucleases (e.g., imidazole, bisimidazole, histamine, imidazole clusters, acridine-imidazole conjugates, Eu3+ complexes of tetraazamacrocycles), dinitrophenyl, HRP, or AP. [00112] Ligands can be proteins, e.g., glycoproteins, or peptides, e.g., molecules having a specific affinity for a co-ligand, or antibodies e.g., an antibody, that binds to a specified cell type such as a cancer cell, endothelial cell, or bone cell. Ligands may also include hormones and hormone receptors. They can also include non-peptidic species, such as lipids, lectins, carbohydrates, vitamins, cofactors, multivalent lactose, multivalent galactose, N-acetyl- galactosamine, N-acetyl-glucosamine multivalent mannose, or multivalent fucose. The ligand can be, for example, a lipopolysaccharide, an activator of p38 MAP kinase, or an activator of NF-κB. [00113] The ligand can be a substance, e.g, a drug, which can increase the uptake of the iRNA agent into the cell, for example, by disrupting the cell's cytoskeleton, e.g., by disrupting the cell's microtubules, microfilaments, and/or intermediate filaments. The drug can be, for example, -37- 4892-3380-3674.1
Atty. Dkt. No.: 115872-2919 taxon, vincristine, vinblastine, cytochalasin, nocodazole, japlakinolide, latrunculin A, phalloidin, swinholide A, indanocine, or myoservin. [00114] In some embodiments, a ligand attached to an iRNA as described herein acts as a pharmacokinetic modulator (PK modulator). PK modulators include lipophiles, bile acids, steroids, phospholipid analogues, peptides, protein binding agents, PEG, vitamins etc. Exemplary PK modulators include, but are not limited to, cholesterol, fatty acids, cholic acid, lithocholic acid, dialkylglycerides, diacylglyceride, phospholipids, sphingolipids, naproxen, ibuprofen, vitamin E, biotin etc. Oligonucleotides that comprise a number of phosphorothioate linkages are also known to bind to serum protein, thus short oligonucleotides, e.g., oligonucleotides of about 5 bases, 10 bases, 15 bases or 20 bases, comprising multiple of phosphorothioate linkages in the backbone are also amenable to the present technology as ligands (e.g., as PK modulating ligands). In addition, aptamers that bind serum components (e.g., serum proteins) are also suitable for use as PK modulating ligands in the embodiments described herein. [00115] Ligand-conjugated oligonucleotides of the present technology may be synthesized by the use of an oligonucleotide that bears a pendant reactive functionality, such as that derived from the attachment of a linking molecule onto the oligonucleotide. This reactive oligonucleotide may be reacted directly with commercially-available ligands, ligands that are synthesized bearing any of a variety of protecting groups, or ligands that have a linking moiety attached thereto. [00116] The oligonucleotides used in the conjugates of the present technology may be conveniently and routinely made through the well-known technique of solid-phase synthesis. Equipment for such synthesis is sold by several vendors including, for example, Applied Biosystems (Foster City, Calif.). Any other means for such synthesis known in the art may additionally or alternatively be employed. It is also known to use similar techniques to prepare other oligonucleotides, such as the phosphorothioates and alkylated derivatives. [00117] In the ligand-conjugated oligonucleotides and ligand-molecule bearing sequence- specific linked nucleosides of the present technology, the oligonucleotides and oligonucleosides -38- 4892-3380-3674.1
Atty. Dkt. No.: 115872-2919 may be assembled on a suitable DNA synthesizer utilizing standard nucleotide or nucleoside precursors, or nucleotide or nucleoside conjugate precursors that already bear the linking moiety, ligand-nucleotide or nucleoside-conjugate precursors that already bear the ligand molecule, or non-nucleoside ligand-bearing building blocks. [00118] When using nucleotide-conjugate precursors that already bear a linking moiety, the synthesis of the sequence-specific linked nucleosides is typically completed, and the ligand molecule is then reacted with the linking moiety to form the ligand-conjugated oligonucleotide. In some embodiments, the oligonucleotides or linked nucleosides of the present technology are synthesized by an automated synthesizer using phosphoramidites derived from ligand-nucleoside conjugates in addition to the standard phosphoramidites and non-standard phosphoramidites that are commercially available and routinely used in oligonucleotide synthesis. [00119] In another aspect, the ligand is a cell-permeation agent, such as a helical cell- permeation agent. In one embodiment, the agent is amphipathic. An exemplary agent is a peptide such as tat or antennopedia. If the agent is a peptide, it can be modified, including a peptidylmimetic, invertomers, non-peptide or pseudo-peptide linkages, and use of D-amino acids. The helical agent is typically an α-helical agent, and can have a lipophilic and a lipophobic phase. [00120] An RGD peptide moiety can be used to target a particular cell type, e.g., a tumor cell, such as an endothelial tumor cell or a breast cancer tumor cell (Zitzmann et al., Cancer Res., 62:5139-43, 2002). An RGD peptide can facilitate targeting of an dsRNA agent to tumors of a variety of other tissues, including the lung, kidney, spleen, or liver (Aoki et al., Cancer Gene Therapy 8:783-787, 2001). Typically, the RGD peptide will facilitate targeting of an iRNA agent to the kidney. The RGD peptide can be linear or cyclic, and can be modified, e.g., glycosylated or methylated to facilitate targeting to specific tissues. For example, a glycosylated RGD peptide can deliver a iRNA agent to a tumor cell expressing α
vβ
3 (Haubner et al., Jour. Nucl. Med., 42:326-336, 2001). [00121] A “cell permeation peptide” is capable of permeating a cell, e.g., a microbial cell, such as a bacterial or fungal cell, or a mammalian cell, such as a human cell. A microbial cell- -39- 4892-3380-3674.1
Atty. Dkt. No.: 115872-2919 permeating peptide can be, for example, an α-helical linear peptide (e.g., LL-37 or Ceropin P1), a disulfide bond-containing peptide (e.g., α-defensin, β-defensin or bactenecin), or a peptide containing only one or two dominating amino acids (e.g., PR-39 or indolicidin). A cell permeation peptide can also include a nuclear localization signal (NLS). For example, a cell permeation peptide can be a bipartite amphipathic peptide, such as MPG, which is derived from the fusion peptide domain of HIV-1 gp41 and the NLS of SV40 large T antigen (Simeoni et al., Nucl. Acids Res.31:2717-2724, 2003). [00122] Linkers [00123] In some embodiments, the conjugate or ligand described herein can be attached to an iRNA oligonucleotide with various linkers that can be cleavable or non-cleavable. [00124] The term “linker” or “linking group” means an organic moiety that connects two parts of a compound, e.g., covalently attaches two parts of a compound. Linkers typically comprise a direct bond or an atom such as oxygen or sulfur, a unit such as C(O), C(O)NH, SO, SO
2, SO
2NH or a chain of atoms, such as, but not limited to, substituted or unsubstituted alkyl, substituted or unsubstituted alkenyl, substituted or unsubstituted alkynyl, arylalkyl, arylalkenyl, arylalkynyl, heteroarylalkyl, heteroarylalkenyl, heteroarylalkynyl, heterocyclylalkyl, heterocyclylalkenyl, heterocyclylalkynyl, aryl, heteroaryl, heterocyclyl, cycloalkyl, cycloalkenyl, alkylarylalkyl, alkylarylalkenyl, alkylarylalkynyl, alkenylarylalkyl, alkenylarylalkenyl, alkenylarylalkynyl, alkynylarylalkyl, alkynylarylalkenyl, alkynylarylalkynyl, alkylheteroarylalkyl, alkylheteroarylalkenyl, alkylheteroarylalkynyl, alkenylheteroarylalkyl, alkenylheteroarylalkenyl, alkenylheteroarylalkynyl, alkynylheteroarylalkyl, alkynylheteroarylalkenyl, alkynylheteroarylalkynyl, alkylheterocyclylalkyl, alkylheterocyclylalkenyl, alkylhererocyclylalkynyl, alkenylheterocyclylalkyl, alkenylheterocyclylalkenyl, alkenylheterocyclylalkynyl, alkynylheterocyclylalkyl, alkynylheterocyclylalkenyl, alkynylheterocyclylalkynyl, alkylaryl, alkenylaryl, alkynylaryl, alkylheteroaryl, alkenylheteroaryl, alkynylhereroaryl, which one or more methylenes can be interrupted or terminated by O, S, S(O), SO
2, N(R8), C(O), substituted or unsubstituted aryl, substituted or unsubstituted heteroaryl, substituted or unsubstituted heterocyclic; where R8 is hydrogen, acyl, -40- 4892-3380-3674.1
Atty. Dkt. No.: 115872-2919 aliphatic or substituted aliphatic. In one embodiment, the linker is between about 1-24 atoms, 2- 24, 3-24, 4-24, 5-24, 6-24, 6-18, 7-18, 8-18 atoms, 7-17, 8-17, 6-16, 7-16, or 8-16 atoms. Exemplary linkers may comprise redox cleavable linking groups, phosphate-based cleavable linking groups, acid cleavable linking groups, ester-based cleavable linking groups, or peptide- based cleavable linking groups. [00125] Delivery of iRNA [00126] The delivery of an iRNA to a subject in need thereof can be achieved in a number of different ways. In vivo delivery can be performed directly by administering a composition comprising an iRNA, e.g., a dsRNA, to a subject. Alternatively, delivery can be performed indirectly by administering one or more vectors that encode and direct the expression of the iRNA. [00127] Direct Delivery [00128] In general, any method of delivering a nucleic acid molecule can be adapted for use with an iRNA (see e.g., Akhtar S. and Julian R L. (1992) Trends Cell. Biol.2(5):139-144 and WO94/02595, which are incorporated herein by reference in their entireties). However, there are three factors that are important to consider in order to successfully deliver an iRNA molecule in vivo: (a) biological stability of the delivered molecule, (2) preventing non-specific effects, and (3) accumulation of the delivered molecule in the target tissue. The non-specific effects of an iRNA can be minimized by local administration, for example by direct injection or implantation into a tissue (as a non-limiting example, a tumor) or topically administering the preparation. Local administration to a treatment site maximizes local concentration of the agent, limits the exposure of the agent to systemic tissues that may otherwise be harmed by the agent or that may degrade the agent, and permits a lower total dose of the iRNA molecule to be administered. Several studies have shown successful knockdown of gene products when an iRNA is administered locally. For example, intraocular delivery of a VEGF dsRNA by intravitreal injection in cynomolgus monkeys (Tolentino, M J., et al (2004) Retina 24:132-138) and subretinal injections in mice (Reich, S J., et al (2003) Mol. Vis.9:210-216) were both shown to prevent neovascularization in an experimental model of age-related macular degeneration. In -41- 4892-3380-3674.1
Atty. Dkt. No.: 115872-2919 addition, direct intratumoral injection of a dsRNA in mice reduces tumor volume (Pille, J., et al (2005) Mol. Ther.11:267-274) and can prolong survival of tumor-bearing mice (Kim, W J., et al (2006) Mol. Ther.14:343-350; Li, S., et al (2007) Mol. Ther.15:515-523). RNA interference has also shown success with local delivery to the CNS by direct injection (Dorn, G., et al. (2004) Nucleic Acids 32:e49; Tan, P H., et al (2005) Gene Ther.12:59-66; Makimura, H., et al (2002) BMC Neurosci.3:18; Shishkina, G T., et al (2004) Neuroscience 129:521-528; Thakker, E R., et al (2004) Proc. Natl. Acad. Sci. U.S.A.101:17270-17275; Akaneya, Y., et al (2005) J. Neurophysiol.93:594-602) and to the lungs by intranasal administration (Howard, K A., et al (2006) Mol. Ther.14:476-484; Zhang, X., et al (2004) J. Biol. Chem.279:10677-10684; Bitko, V., et al (2005) Nat. Med.11:50-55). For administering an iRNA systemically for the treatment of a disease, the RNA can be modified or alternatively delivered using a drug delivery system; both methods act to prevent the rapid degradation of the dsRNA by endo- and exo-nucleases in vivo. [00129] Modification of the RNA or the pharmaceutical carrier can also permit targeting of the iRNA composition to the target tissue and avoid undesirable off-target effects. iRNA molecules can be modified by chemical conjugation to other groups, e.g., a lipid or carbohydrate group as described herein. Such conjugates can be used to target iRNA to particular cells, e.g., liver cells, e.g., hepatocytes. For example, GalNAc conjugates or lipid (e.g., LNP) formulations can be used to target iRNA to particular cells, e.g., liver cells, e.g., hepatocytes. [00130] Lipophilic groups such as cholesterol to enhance cellular uptake and prevent degradation. For example, an iRNA directed against ApoB conjugated to a lipophilic cholesterol moiety was injected systemically into mice and resulted in knockdown of apoB mRNA in both the liver and jejunum (Soutschek, J., et al (2004) Nature 432:173-178). Conjugation of an iRNA to an aptamer has been shown to inhibit tumor growth and mediate tumor regression in a mouse model of prostate cancer (McNamara, J O., et al (2006) Nat. Biotechnol.24:1005-1015). In an alternative embodiment, the iRNA can be delivered using drug delivery systems such as a nanoparticle, a dendrimer, a polymer, liposomes, or a cationic delivery system. Positively charged cationic delivery systems facilitate binding of an iRNA molecule (negatively charged) -42- 4892-3380-3674.1
Atty. Dkt. No.: 115872-2919 and also enhance interactions at the negatively charged cell membrane to permit efficient uptake of an iRNA by the cell. Cationic lipids, dendrimers, or polymers can either be bound to an iRNA, or induced to form a vesicle or micelle (see e.g., Kim S H., et al (2008) Journal of Controlled Release 129(2):107-116) that encases an iRNA. The formation of vesicles or micelles further prevents degradation of the iRNA when administered systemically. Methods for making and administering cationic-iRNA complexes are well within the abilities of one skilled in the art (see e.g., Sorensen, D R., et al (2003) J. Mol. Biol.327:761-766; Verma, U N., et al (2003) Clin. Cancer Res.9:1291-1300; Arnold, A S et al (2007) J. Hypertens.25:197-205, which are incorporated herein by reference in their entirety). Some non-limiting examples of drug delivery systems useful for systemic delivery of iRNAs include DOTAP (Sorensen, D R., et al (2003), supra; Verma, U N., et al (2003), supra), Oligofectamine, “solid nucleic acid lipid particles” (Zimmermann, T S., et al (2006) Nature 441:111-114), cardiolipin (Chien, P Y., et al (2005) Cancer Gene Ther.12:321-328; Pal, A., et al (2005) Int J. Oncol.26:1087-1091), polyethyleneimine (Bonnet M E., et al (2008) Pharm. Res. August 16 Epub ahead of print; Aigner, A. (2006) J. Biomed. Biotechnol.71659), Arg-Gly-Asp (RGD) peptides (Liu, S. (2006) Mol. Pharm.3:472-487), and polyamidoamines (Tomalia, D A., et al (2007) Biochem. Soc. Trans.35:61-67; Yoo, H., et al (1999) Pharm. Res.16:1799-1804). In some embodiments, an iRNA forms a complex with cyclodextrin for systemic administration. Methods for administration and pharmaceutical compositions of iRNAs and cyclodextrins can be found in U.S. Pat. No.7,427,605, which is herein incorporated by reference in its entirety. [00131] Vector Encoded iRNAs [00132] In another aspect, iRNA targeting the disease-inducing gene can be expressed from transcription units inserted into DNA or RNA vectors (see, e.g., Couture, A, et al., TIG. (1996), 12:5-10; Skillern, A., et al., International PCT Publication No. WO 00/22113, Conrad, International PCT Publication No. WO 00/22114, and Conrad, U.S. Pat. No.6,054,299). Expression can be transient (on the order of hours to weeks) or sustained (weeks to months or longer), depending upon the specific construct used and the target tissue or cell type. These transgenes can be introduced as a linear construct, a circular plasmid, or a viral vector, which can -43- 4892-3380-3674.1
Atty. Dkt. No.: 115872-2919 be an integrating or non-integrating vector. The transgene can also be constructed to permit it to be inherited as an extrachromosomal plasmid (Gassmann, et al., Proc. Natl. Acad. Sci. USA (1995) 92:1292). [00133] The individual strand or strands of an iRNA can be transcribed from a promoter on an expression vector. Where two separate strands are to be expressed to generate, for example, a dsRNA, two separate expression vectors can be co-introduced (e.g., by transfection or infection) into a target cell. Alternatively each individual strand of a dsRNA can be transcribed by promoters both of which are located on the same expression plasmid. In one embodiment, a dsRNA is expressed as an inverted repeat joined by a linker polynucleotide sequence such that the dsRNA has a stem and loop structure. [00134] An iRNA expression vector is typically a DNA plasmid or viral vector. An expression vector compatible with eukaryotic cells, e.g., with vertebrate cells, can be used to produce recombinant constructs for the expression of an iRNA as described herein. Eukaryotic cell expression vectors are well known in the art and are available from a number of commercial sources. Typically, such vectors contain convenient restriction sites for insertion of the desired nucleic acid segment. Delivery of iRNA expressing vectors can be systemic, such as by intravenous or intramuscular administration, by administration to target cells ex-planted from the patient followed by reintroduction into the patient, or by any other means that allows for introduction into a desired target cell. [00135] An iRNA expression plasmid can be transfected into a target cell as a complex with a cationic lipid carrier (e.g., Oligofectamine) or a non-cationic lipid-based carrier (e.g., Transit- TKO™). Multiple lipid transfections for iRNA-mediated knockdowns targeting different regions of a target RNA over a period of a week or more are also contemplated by the present technology. Successful introduction of vectors into host cells can be monitored using various known methods. For example, transient transfection can be signaled with a reporter, such as a fluorescent marker, such as Green Fluorescent Protein (GFP). Stable transfection of cells ex vivo can be ensured using markers that provide the transfected cell with resistance to specific environmental factors (e.g., antibiotics and drugs), such as hygromycin B resistance. -44- 4892-3380-3674.1
Atty. Dkt. No.: 115872-2919 [00136] Viral vector systems which can be utilized with the methods and compositions described herein include, but are not limited to, (a) adenovirus vectors; (b) retrovirus vectors, including but not limited to lentiviral vectors, moloney murine leukemia virus, etc.; (c) adeno- associated virus vectors; (d) herpes simplex virus vectors; (e) SV40 vectors; (f) polyoma virus vectors; (g) papilloma virus vectors; (h) picornavirus vectors; (i) pox virus vectors such as an orthopox, e.g., vaccinia virus vectors or avipox, e.g., canary pox or fowl pox; and (j) a helper- dependent or gutless adenovirus. Replication-defective viruses can also be advantageous. Different vectors will or will not become incorporated into the cells' genome. The constructs can include viral sequences for transfection, if desired. Alternatively, the construct may be incorporated into vectors capable of episomal replication, e.g EPV and EBV vectors. Constructs for the recombinant expression of an iRNA will generally require regulatory elements, e.g., promoters, enhancers, etc., to ensure the expression of the iRNA in target cells. Other aspects to consider for vectors and constructs are further described below. [00137] Vectors useful for the delivery of an iRNA will include regulatory elements (promoter, enhancer, etc.) sufficient for expression of the iRNA in the desired target cell or tissue. The regulatory elements can be chosen to provide either constitutive or regulated/inducible expression. [00138] Expression of the iRNA can be precisely regulated, for example, by using an inducible regulatory sequence that is sensitive to certain physiological regulators, e.g., circulating glucose levels, or hormones (Docherty et al., 1994, FASEB J.8:20-24). Such inducible expression systems, suitable for the control of dsRNA expression in cells or in mammals include, for example, regulation by ecdysone, by estrogen, progesterone, tetracycline, chemical inducers of dimerization, and isopropyl-β-D1-thiogalactopyranoside (IPTG). A person skilled in the art would be able to choose the appropriate regulatory/promoter sequence based on the intended use of the iRNA transgene. [00139] In a specific embodiment, viral vectors that contain nucleic acid sequences encoding an iRNA can be used. For example, a retroviral vector can be used (see Miller et al., Meth. Enzymol.217:581-599 (1993)). These retroviral vectors contain the components necessary for -45- 4892-3380-3674.1
Atty. Dkt. No.: 115872-2919 the correct packaging of the viral genome and integration into the host cell DNA. The nucleic acid sequences encoding an iRNA are cloned into one or more vectors, which facilitates delivery of the nucleic acid into a patient. More detail about retroviral vectors can be found, for example, in Boesen et al., Biotherapy 6:291-302 (1994), which describes the use of a retroviral vector to deliver the mdr1 gene to hematopoietic stem cells in order to make the stem cells more resistant to chemotherapy. Other references illustrating the use of retroviral vectors in gene therapy are: Clowes et al., J. Clin. Invest.93:644-651 (1994); Kiem et al., Blood 83:1467-1473 (1994); Salmons and Gunzberg, Human Gene Therapy 4:129-141 (1993); and Grossman and Wilson, Curr. Opin. in Genetics and Devel.3:110-114 (1993). Lentiviral vectors contemplated for use include, for example, the HIV based vectors described in U.S. Pat. Nos.6,143,520; 5,665,557; and 5,981,276, which are herein incorporated by reference. [00140] Adenoviruses are also contemplated for use in delivery of iRNAs. Adenoviruses are especially attractive vehicles, e.g., for delivering genes to respiratory epithelia. Adenoviruses naturally infect respiratory epithelia where they cause a mild disease. Other targets for adenovirus-based delivery systems are liver, the central nervous system, endothelial cells, and muscle. Adenoviruses have the advantage of being capable of infecting non-dividing cells. Kozarsky and Wilson, Current Opinion in Genetics and Development 3:499-503 (1993) present a review of adenovirus-based gene therapy. Bout et al., Human Gene Therapy 5:3-10 (1994) demonstrated the use of adenovirus vectors to transfer genes to the respiratory epithelia of rhesus monkeys. Other instances of the use of adenoviruses in gene therapy can be found in Rosenfeld et al., Science 252:431-434 (1991); Rosenfeld et al., Cell 68:143-155 (1992); Mastrangeli et al., J. Clin. Invest.91:225-234 (1993); PCT Publication WO94/12649; and Wang, et al., Gene Therapy 2:775-783 (1995). A suitable AV vector for expressing an iRNA featured in the present disclosure, a method for constructing the recombinant AV vector, and a method for delivering the vector into target cells, are described in Xia H et al. (2002), Nat. Biotech.20: 1006-1010. [00141] Use of Adeno-associated virus (AAV) vectors is also contemplated (Walsh et al., Proc. Soc. Exp. Biol. Med.204:289-300 (1993); U.S. Pat. No.5,436,146). In one embodiment, the iRNA can be expressed as two separate, complementary single-stranded RNA molecules -46- 4892-3380-3674.1
Atty. Dkt. No.: 115872-2919 from a recombinant AAV vector having, for example, either the U6 or H1 RNA promoters, or the cytomegalovirus (CMV) promoter. Suitable AAV vectors for expressing the dsRNA featured in the present technology, methods for constructing the recombinant AV vector, and methods for delivering the vectors into target cells are described in Samulski R et al. (1987), J. Virol.61: 3096-3101; Fisher K J et al. (1996), J. Virol, 70: 520-532; Samulski R et al. (1989), J. Virol.63: 3822-3826; U.S. Pat. No.5,252,479; U.S. Pat. No.5,139,941; International Patent Application No. WO 94/13788; and International Patent Application No. WO 93/24641, the entire disclosures of which are herein incorporated by reference. [00142] Another typical viral vector is a pox virus such as a vaccinia virus, for example an attenuated vaccinia such as Modified Virus Ankara (MVA) or NYVAC, an avipox such as fowl pox or canary pox. [00143] The tropism of viral vectors can be modified by pseudotyping the vectors with envelope proteins or other surface antigens from other viruses, or by substituting different viral capsid proteins, as appropriate. For example, lentiviral vectors can be pseudotyped with surface proteins from vesicular stomatitis virus (VSV), rabies, Ebola, Mokola, and the like. AAV vectors can be made to target different cells by engineering the vectors to express different capsid protein serotypes; see, e.g., Rabinowitz J E et al. (2002), J Virol 76:791-801, the entire disclosure of which is herein incorporated by reference. [00144] The pharmaceutical preparation of a vector can include the vector in an acceptable diluent, or can include a slow release matrix in which the gene delivery vehicle is imbedded. Alternatively, where the complete gene delivery vector can be produced intact from recombinant cells, e.g., retroviral vectors, the pharmaceutical preparation can include one or more cells which produce the gene delivery system. [00145] Liposomal Formulations [00146] There are many organized surfactant structures besides microemulsions that have been studied and used for the formulation of drugs. These include monolayers, micelles, bilayers and vesicles. Vesicles, such as liposomes, have attracted great interest because of their specificity and the duration of action they offer from the standpoint of drug delivery. As used in -47- 4892-3380-3674.1
Atty. Dkt. No.: 115872-2919 the present technology, the term “liposome” means a vesicle composed of amphiphilic lipids arranged in a spherical bilayer or bilayers. [00147] Liposomes are unilamellar or multilamellar vesicles which have a membrane formed from a lipophilic material and an aqueous interior. The aqueous portion contains the composition to be delivered. Cationic liposomes possess the advantage of being able to fuse to the cell wall. Non-cationic liposomes, although not able to fuse as efficiently with the cell wall, are taken up by macrophages in vivo. [00148] In order to traverse intact mammalian skin, lipid vesicles must pass through a series of fine pores, each with a diameter less than 50 nm, under the influence of a suitable transdermal gradient. Therefore, it is desirable to use a liposome which is highly deformable and able to pass through such fine pores. [00149] Further advantages of liposomes include; liposomes obtained from natural phospholipids are biocompatible and biodegradable; liposomes can incorporate a wide range of water and lipid soluble drugs; liposomes can protect encapsulated drugs in their internal compartments from metabolism and degradation (Rosoff, in Pharmaceutical Dosage Forms, Lieberman, Rieger and Banker (Eds.), 1988, Marcel Dekker, Inc., New York, N.Y., volume 1, p. 245). Important considerations in the preparation of liposome formulations are the lipid surface charge, vesicle size and the aqueous volume of the liposomes. [00150] Liposomes are useful for the transfer and delivery of active ingredients to the site of action. Because the liposomal membrane is structurally similar to biological membranes, when liposomes are applied to a tissue, the liposomes start to merge with the cellular membranes and as the merging of the liposome and cell progresses, the liposomal contents are emptied into the cell where the active agent may act. [00151] Liposomal formulations have been the focus of extensive investigation as the mode of delivery for many drugs. There is growing evidence that for topical administration, liposomes present several advantages over other formulations. Such advantages include reduced side- effects related to high systemic absorption of the administered drug, increased accumulation of -48- 4892-3380-3674.1
Atty. Dkt. No.: 115872-2919 the administered drug at the desired target, and the ability to administer a wide variety of drugs, both hydrophilic and hydrophobic, into the skin. [00152] Several reports have detailed the ability of liposomes to deliver agents including high- molecular weight DNA into the skin. Compounds including analgesics, antibodies, hormones and high-molecular weight DNAs have been administered to the skin. The majority of applications resulted in the targeting of the upper epidermis. [00153] Liposomes fall into two broad classes. Cationic liposomes are positively charged liposomes which interact with the negatively charged DNA molecules to form a stable complex. The positively charged DNA/liposome complex binds to the negatively charged cell surface and is internalized in an endosome. Due to the acidic pH within the endosome, the liposomes are ruptured, releasing their contents into the cell cytoplasm (Wang et al., Biochem. Biophys. Res. Commun., 1987, 147, 980-985). [00154] Liposomes which are pH-sensitive or negatively-charged, entrap DNA rather than complex with it. Since both the DNA and the lipid are similarly charged, repulsion rather than complex formation occurs. Nevertheless, some DNA is entrapped within the aqueous interior of these liposomes. pH-sensitive liposomes have been used to deliver DNA encoding the thymidine kinase gene to cell monolayers in culture. Expression of the exogenous gene was detected in the target cells (Zhou et al., Journal of Controlled Release, 1992, 19, 269-274). [00155] One major type of liposomal composition includes phospholipids other than naturally-derived phosphatidylcholine. Neutral liposome compositions, for example, can be formed from dimyristoyl phosphatidylcholine (DMPC) or dipalmitoyl phosphatidylcholine (DPPC). Anionic liposome compositions generally are formed from dimyristoyl phosphatidylglycerol, while anionic fusogenic liposomes are formed primarily from dioleoyl phosphatidylethanolamine (DOPE). Another type of liposomal composition is formed from phosphatidylcholine (PC) such as, for example, soybean PC, and egg PC. Another type is formed from mixtures of phospholipid and/or phosphatidylcholine and/or cholesterol. [00156] Several studies have assessed the topical delivery of liposomal drug formulations to the skin. Application of liposomes containing interferon to guinea pig skin resulted in a -49- 4892-3380-3674.1
Atty. Dkt. No.: 115872-2919 reduction of skin herpes sores while delivery of interferon via other means (e.g., as a solution or as an emulsion) were ineffective (Weiner et al., Journal of Drug Targeting, 1992, 2, 405-410). Further, an additional study tested the efficacy of interferon administered as part of a liposomal formulation to the administration of interferon using an aqueous system, and concluded that the liposomal formulation was superior to aqueous administration (du Plessis et al., Antiviral Research, 1992, 18, 259-265). [00157] Non-ionic liposomal systems have also been examined to determine their utility in the delivery of drugs to the skin, in particular systems comprising non-ionic surfactant and cholesterol. Non-ionic liposomal formulations comprising Novasome™ I (glyceryl dilaurate/cholesterol/polyoxyethylene-10-stearyl ether) and Novasome™ II (glyceryl distearate/cholesterol/polyoxyethylene-10-stearyl ether) were used to deliver cyclosporin-A into the dermis of mouse skin. Results indicated that such non-ionic liposomal systems were effective in facilitating the deposition of cyclosporin-A into different layers of the skin (Hu et al. S. T. P. Pharma. Sci., 1994, 4, 6, 466). [00158] Liposomes also include “sterically stabilized” liposomes, a term which, as used herein, refers to liposomes comprising one or more specialized lipids that, when incorporated into liposomes, result in enhanced circulation lifetimes relative to liposomes lacking such specialized lipids. Examples of sterically stabilized liposomes are those in which part of the vesicle-forming lipid portion of the liposome (A) comprises one or more glycolipids, such as monosialoganglioside G
M1, or (B) is derivatized with one or more hydrophilic polymers, such as a polyethylene glycol (PEG) moiety. While not wishing to be bound by any particular theory, it is thought in the art that, at least for sterically stabilized liposomes containing gangliosides, sphingomyelin, or PEG-derivatized lipids, the enhanced circulation half-life of these sterically stabilized liposomes derives from a reduced uptake into cells of the reticuloendothelial system (RES) (Allen et al., FEBS Letters, 1987, 223, 42; Wu et al., Cancer Research, 1993, 53, 3765). [00159] Various liposomes comprising one or more glycolipids are known in the art. Papahadjopoulos et al. (Ann. N.Y. Acad. Sci., 1987, 507, 64) reported the ability of monosialoganglioside G
M1, galactocerebroside sulfate and phosphatidylinositol to improve blood -50- 4892-3380-3674.1
Atty. Dkt. No.: 115872-2919 half-lives of liposomes. These findings were expounded upon by Gabizon et al. (Proc. Natl. Acad. Sci. U.S.A., 1988, 85, 6949). U.S. Pat. No.4,837,028 and WO 88/04924, both to Allen et al., disclose liposomes comprising (1) sphingomyelin and (2) the ganglioside G
M1 or a galactocerebroside sulfate ester. U.S. Pat. No.5,543,152 (Webb et al.) discloses liposomes comprising sphingomyelin. Liposomes comprising 1,2-sn-dimyristoylphosphatidylcholine are disclosed in WO 97/13499 (Lim et al). [00160] Many liposomes comprising lipids derivatized with one or more hydrophilic polymers, and methods of preparation thereof, are known in the art. Sunamoto et al. (Bull. Chem. Soc. Jpn., 1980, 53, 2778) described liposomes comprising a nonionic detergent, 2C
1215G, that contains a PEG moiety. Illum et al. (FEBS Lett., 1984, 167, 79) noted that hydrophilic coating of polystyrene particles with polymeric glycols results in significantly enhanced blood half-lives. Synthetic phospholipids modified by the attachment of carboxylic groups of polyalkylene glycols (e.g., PEG) are described by Sears (U.S. Pat. Nos.4,426,330 and 4,534,899). Klibanov et al. (FEBS Lett., 1990, 268, 235) described experiments demonstrating that liposomes comprising phosphatidylethanolamine (PE) derivatized with PEG or PEG stearate have significant increases in blood circulation half-lives. Blume et al. (Biochimica et Biophysica Acta, 1990, 1029, 91) extended such observations to other PEG-derivatized phospholipids, e.g., DSPE-PEG, formed from the combination of distearoylphosphatidylethanolamine (DSPE) and PEG. Liposomes having covalently bound PEG moieties on their external surface are described in European Patent No. EP 0445131 B1 and WO 90/04384 to Fisher. Liposome compositions containing 1-20 mole percent of PE derivatized with PEG, and methods of use thereof, are described by Woodle et al. (U.S. Pat. Nos.5,013,556 and 5,356,633) and Martin et al. (U.S. Pat. No.5,213,804 and European Patent No. EP 0496813 B1). Liposomes comprising a number of other lipid-polymer conjugates are disclosed in WO 91/05545 and U.S. Pat. No.5,225,212 (both to Martin et al.) and in WO 94/20073 (Zalipsky et al.). Liposomes comprising PEG-modified ceramide lipids are described in WO 96/10391 (Choi et al). U.S. Pat. No.5,540,935 (Miyazaki et al.) and U.S. Pat. No.5,556,948 (Tagawa et al.) describe PEG-containing liposomes that can be further derivatized with functional moieties on their surfaces. -51- 4892-3380-3674.1
Atty. Dkt. No.: 115872-2919 [00161] A number of liposomes comprising nucleic acids are known in the art. WO 96/40062 to Thierry et al. discloses methods for encapsulating high molecular weight nucleic acids in liposomes. U.S. Pat. No.5,264,221 to Tagawa et al. discloses protein-bonded liposomes and asserts that the contents of such liposomes may include a dsRNA. U.S. Pat. No.5,665,710 to Rahman et al. describes certain methods of encapsulating oligodeoxynucleotides in liposomes. WO 97/04787 to Love et al. discloses liposomes comprising dsRNAs targeted to the raf gene. [00162] Transfersomes are yet another type of liposomes, and are highly deformable lipid aggregates which are attractive candidates for drug delivery vehicles. Transfersomes may be described as lipid droplets which are so highly deformable that they are easily able to penetrate through pores which are smaller than the droplet. Transfersomes are adaptable to the environment in which they are used, e.g., they are self-optimizing (adaptive to the shape of pores in the skin), self-repairing, frequently reach their targets without fragmenting, and often self- loading. To make transfersomes it is possible to add surface edge-activators, usually surfactants, to a standard liposomal composition. Transfersomes have been used to deliver serum albumin to the skin. The transfersome-mediated delivery of serum albumin has been shown to be as effective as subcutaneous injection of a solution containing serum albumin. [00163] Surfactants find wide application in formulations such as emulsions (including microemulsions) and liposomes. The most common way of classifying and ranking the properties of the many different types of surfactants, both natural and synthetic, is by the use of the hydrophile/lipophile balance (HLB). The nature of the hydrophilic group (also known as the “head”) provides the most useful means for categorizing the different surfactants used in formulations (Rieger, in Pharmaceutical Dosage Forms, Marcel Dekker, Inc., New York, N.Y., 1988, p.285). [00164] If the surfactant molecule is not ionized, it is classified as a nonionic surfactant. Nonionic surfactants find wide application in pharmaceutical and cosmetic products and are usable over a wide range of pH values. In general their HLB values range from 2 to about 18 depending on their structure. Nonionic surfactants include nonionic esters such as ethylene glycol esters, propylene glycol esters, glyceryl esters, polyglyceryl esters, sorbitan esters, sucrose -52- 4892-3380-3674.1
Atty. Dkt. No.: 115872-2919 esters, and ethoxylated esters. Nonionic alkanolamides and ethers such as fatty alcohol ethoxylates, propoxylated alcohols, and ethoxylated/propoxylated block polymers are also included in this class. The polyoxyethylene surfactants are the most popular members of the nonionic surfactant class. [00165] If the surfactant molecule carries a negative charge when it is dissolved or dispersed in water, the surfactant is classified as anionic. Anionic surfactants include carboxylates such as soaps, acyl lactylates, acyl amides of amino acids, esters of sulfuric acid such as alkyl sulfates and ethoxylated alkyl sulfates, sulfonates such as alkyl benzene sulfonates, acyl isethionates, acyl taurates and sulfosuccinates, and phosphates. The most important members of the anionic surfactant class are the alkyl sulfates and the soaps. [00166] If the surfactant molecule carries a positive charge when it is dissolved or dispersed in water, the surfactant is classified as cationic. Cationic surfactants include quaternary ammonium salts and ethoxylated amines. The quaternary ammonium salts are the most used members of this class. If the surfactant molecule has the ability to carry either a positive or negative charge, the surfactant is classified as amphoteric Amphoteric surfactants include acrylic acid derivatives, substituted alkylamides, N-alkylbetaines and phosphatides. The use of surfactants in drug products, formulations and in emulsions has been reviewed (Rieger, in Pharmaceutical Dosage Forms, Marcel Dekker, Inc., New York, N.Y., 1988, p.285). [00167] Nucleic Acid Lipid Particles [00168] In one embodiment, an RNAi agent featured in the present technology is fully encapsulated in the lipid formulation, e.g.., to form a SPLP, pSPLP, SNALP, or other nucleic acid-lipid particle. As used herein, the term “SNALP” refers to a stable nucleic acid-lipid particle, including SPLP. As used herein, the term “SPLP” refers to a nucleic acid-lipid particle comprising plasmid DNA encapsulated within a lipid vesicle. SNALPs and SPLPs typically contain a cationic lipid, a non-cationic lipid, and a lipid that prevents aggregation of the particle (e.g.., a PEG-lipid conjugate). SNALPs and SPLPs are extremely useful for systemic applications, as they exhibit extended circulation lifetimes following intravenous (i.v.) injection and accumulate at distal sites (e.g., sites physically separated from the administration site). -53- 4892-3380-3674.1
Atty. Dkt. No.: 115872-2919 SPLPs include “pSPLP,” which include an encapsulated condensing agent-nucleic acid complex as set forth in PCT Publication No. WO 00/03683. The particles of the present technology typically have a mean diameter of about 50 nm to about 150 nm, more typically about 60 nm to about 130 nm, more typically about 70 nm to about 110 nm, most typically about 70 nm to about 90 nm, and are substantially nontoxic. In addition, the nucleic acids when present in the nucleic acid-lipid particles of the present technology are resistant in aqueous solution to degradation with a nuclease. Nucleic acid-lipid particles and their method of preparation are disclosed in, e.g.., U.S. Pat. Nos.5,976,567; 5,981,501; 6,534,484; 6,586,410; 6,815,432; and PCT Publication No. WO 96/40964. [00169] In one embodiment, the lipid to drug ratio (mass/mass ratio) (e.g.., lipid to dsRNA ratio) will be in the range of from about 1:1 to about 50:1, from about 1:1 to about 25:1, from about 3:1 to about 15:1, from about 4:1 to about 10:1, from about 5:1 to about 9:1, or about 6:1 to about 9:1. [00170] The cationic lipid may be, for example, N,N-dioleyl-N,N-dimethylammonium chloride (DODAC), N,N-distearyl-N,N-dimethylammonium bromide (DDAB), N-(I-(2,3- dioleoyloxy)propyl)-N,N,N-trimethylammonium chloride (DOTAP), N-(I-(2,3- dioleyloxy)propyl)-N,N,N-trimethylammonium chloride (DOTMA), N,N-dimethyl-2,3- dioleyloxy)propylamine (DODMA), 1,2-DiLinoleyloxy-N,N-dimethylaminopropane (DLinDMA),1,2-Dilinolenyloxy-N,N-dimethylaminopropane (DLenDMA), 1,2- Dilinoleylcarbamoyloxy-3-dimethylaminopropane (DLin-C-DAP), 1,2-Dilinoleyoxy-3- (dimethylamino)acetoxypropane (DLin-DAC), 1,2-Dilinoleyoxy-3-morpholinopropane (DLin- MA), 1,2-Dilinoleoyl-3-dimethylaminopropane (DLinDAP), 1,2-Dilinoleylthio-3- dimethylaminopropane (DLin-S-DMA), 1-Linoleoyl-2-linoleyloxy-3-dimethylaminopropane (DLin-2-DMAP), 1,2-Dilinoleyloxy-3-trimethylaminopropane chloride salt (DLin-TMA.Cl), 1,2-Dilinoleoyl-3-trimethylaminopropane chloride salt (DLin-TAP.Cl), 1,2-Dilinoleyloxy-3-(N- methylpiperazino)propane (DLin-MPZ), or 3-(N,N-Dilinoleylamino)-1,2-propanediol (DLinAP), 3-(N,N-Dioleylamino)-1,2-propanedio (DOAP), 1,2-Dilinoleyloxo-3-(2-N,N- dimethylamino)ethoxypropane (DLin-EG-DMA), 1,2-Dilinolenyloxy-N,N- -54- 4892-3380-3674.1
Atty. Dkt. No.: 115872-2919 dimethylaminopropane (DLinDMA), 2,2-Dilinoleyl-4-dimethylaminomethyl-[1,3]-dioxolane (DLin-K-DMA) or analogs thereof, (3aR,5s,6aS)-N,N-dimethyl-2,2-di((9Z,12Z)-octadeca-9,12- dienyl)tetrahydro-3aH-cyclopenta[d][1,3]dioxol-5-amine (ALN100), (6Z,9Z,28Z,31Z)- heptatriaconta-6,9,28,31-tetraen-19-yl 4-(dimethylamino)butanoate (MC3), 1,1′-(2-(4-(2-((2- (bis(2-hydroxydodecyl)amino)ethyl)(2-hydroxydodecyl)amino)ethyl)piperazin-1- yl)ethylazanediyl)didodecan-2-ol (Tech G1), or a mixture thereof. The cationic lipid may comprise from about 20 mol % to about 50 mol % or about 40 mol % of the total lipid present in the particle. [00171] In another embodiment, the compound 2,2-Dilinoleyl-4-dimethylaminoethyl-[1,3]- dioxolane can be used to prepare lipid-siRNA nanoparticles. Synthesis of 2,2-Dilinoleyl-4- dimethylaminoethyl-[1,3]-dioxolane is described in U.S. provisional patent application No. 61/107,998 filed on Oct.23, 2008, which is herein incorporated by reference. [00172] In one embodiment, the lipid-siRNA particle includes 40% 2,2-Dilinoleyl-4- dimethylaminoethyl-[1,3]-dioxolane: 10% DSPC: 40% Cholesterol: 10% PEG-C-DOMG (mole percent) with a particle size of 63.0±20 nm and a 0.027 siRNA/Lipid Ratio. [00173] The non-cationic lipid may be an anionic lipid or a neutral lipid including, but not limited to, distearoylphosphatidylcholine (DSPC), dioleoylphosphatidylcholine (DOPC), dipalmitoylphosphatidylcholine (DPPC), dioleoylphosphatidylglycerol (DOPG), dipalmitoylphosphatidylglycerol (DPPG), dioleoyl-phosphatidylethanolamine (DOPE), palmitoyloleoylphosphatidylcholine (POPC), palmitoyloleoylphosphatidylethanolamine (POPE), dioleoyl-phosphatidylethanolamine 4-(N-maleimidomethyl)-cyclohexane-1-carboxylate (DOPE- mal), dipalmitoyl phosphatidyl ethanolamine (DPPE), dimyristoylphosphoethanolamine (DMPE), distearoyl-phosphatidyl-ethanolamine (DSPE), 16-O-monomethyl PE, 16-O-dimethyl PE, 18-1-trans PE, 1-stearoyl-2-oleoyl-phosphatidyethanolamine (SOPE), cholesterol, or a mixture thereof. The non-cationic lipid may be from about 5 mol % to about 90 mol %, about 10 mol %, or about 58 mol % if cholesterol is included, of the total lipid present in the particle. [00174] The conjugated lipid that inhibits aggregation of particles may be, for example, a polyethyleneglycol (PEG)-lipid including, without limitation, a PEG-diacylglycerol (DAG), a -55- 4892-3380-3674.1
Atty. Dkt. No.: 115872-2919 PEG-dialkyloxypropyl (DAA), a PEG-phospholipid, a PEG-ceramide (Cer), or a mixture thereof. The PEG-DAA conjugate may be, for example, a PEG-dilauryloxypropyl (Ci
2), a PEG- dimyristyloxypropyl (Ci
4), a PEG-dipalmityloxypropyl (Ci
6), or a PEG-distearyloxypropyl (C]
8). The conjugated lipid that prevents aggregation of particles may be from 0 mol % to about 20 mol % or about 2 mol % of the total lipid present in the particle. [00175] In some embodiments, the nucleic acid-lipid particle further includes cholesterol at, e.g.., about 10 mol % to about 60 mol % or about 48 mol % of the total lipid present in the particle. [00176] In some embodiments, the iRNA is formulated in a lipid nanoparticle (LNP). ALAS1/ALAS2-specific Inhibitory Nucleic Acids of the Present Technology [00177] As used herein, “ALAS1” (also known as ALAS-1; δ-aminolevulinate synthase 1; δ- ALA synthase 1; 5′-aminolevulinic acid synthase 1; ALAS-H; ALASH; ALAS-N; ALAS3; EC2.3.1.37; 5-aminolevulinate synthase, nonspecific, mitochondrial; ALAS; MIG4; OTTHUMP00000212619; OTTHUMP00000212620; OTTHUMP00000212621; OTTHUMP00000212622; migration-inducing protein 4; EC 2.3.1) refers to a nuclear-encoded mitochondrial enzyme that is the first and typically rate-limiting enzyme in the mammalian heme biosynthetic pathway. ALAS1 catalyzes the condensation of glycine with succinyl-CoA to form δ-aminolevulinic acid (ALA). The human ALAS1 gene is expressed ubiquitously, is found on chromosome 3p21.1 and typically encodes a sequence of 640 amino acids. [00178] In contrast, the ALAS-2 gene, which encodes an isozyme, is found on chromosome Xp11.21, and typically encodes a sequence of 550 amino acids. While it has long been believed that ALAS2 expression is erythroid-specific, our recent unpublished studies show that murine hepatic cells express Alas2 mRNA in addition to Alas1 mRNA. As used herein an “ALAS1 protein” means any protein variant of ALAS1 from any species (e.g., human, mouse, non-human primate), as well as any mutants and fragments thereof that retain an ALAS1 activity. Similarly, an “ALAS1 transcript” refers to any transcript variant of ALAS1, from any species (e.g., human, mouse, non-human primate). The level of the mature encoded ALAS1 protein is regulated by heme: high levels of heme down-regulate the mature enzyme in mitochondria while low heme -56- 4892-3380-3674.1
Atty. Dkt. No.: 115872-2919 levels up-regulate. Multiple alternatively spliced variants, encoding the same protein, have been identified. [00179] Exemplary nucleic acid sequences of human ALAS1 and ALAS2 transcript variants, represented by SEQ ID NOs: 1-7 are provided below: [00180] NM_000688.6 Homo sapiens 5'-aminolevulinate synthase 1 (ALAS1), transcript variant 1, mRNA; nuclear gene for mitochondrial product (SEQ ID NO: 1) AGGCTGCTCCCGGACAAGGGCAACGAGCGTTTCGTTTGGACTTCTCGACTTGAGTGCCCGCCTCCTTCGC CGCCGCCTCTGCAGTCCTCAGCGCAGTTATGCCCAGTTCTTCCCGCTGTGGGGACACGACCACGGAGGAA TCCTTGCTTCAGGGACTCGGGACCCTGCTGGACCCCTTCCTCGGGTTTAGGGGATGTGGGGACCAGGAGA AAGTCAGGATCCCTAAGAGTCTTCCCTGCCTGGATGGATGAGTGGCTTCTTCTCCACCTAGATTCTTTCC ACAGGAGCCAGCATACTTCCTGAACATGGAGAGTGTTGTTCGCCGCTGCCCATTCTTATCCCGAGTCCCC CAGGCCTTTCTGCAGAAAGCAGGCAAATCTCTGTTGTTCTATGCCCAAAACTGCCCCAAGATGATGGAAG TTGGGGCCAAGCCAGCCCCTCGGGCATTGTCCACTGCAGCAGTACACTACCAACAGATCAAAGAAACCCC TCCGGCCAGTGAGAAAGACAAAACTGCTAAGGCCAAGGTCCAACAGACTCCTGATGGATCCCAGCAGAGT CCAGATGGCACACAGCTTCCGTCTGGACACCCCTTGCCTGCCACAAGCCAGGGCACTGCAAGCAAATGCC CTTTCCTGGCAGCACAGATGAATCAGAGAGGCAGCAGTGTCTTCTGCAAAGCCAGTCTTGAGCTTCAGGA GGATGTGCAGGAAATGAATGCCGTGAGGAAAGAGGTTGCTGAAACCTCAGCAGGCCCCAGTGTGGTTAGT GTGAAAACCGATGGAGGGGATCCCAGTGGACTGCTGAAGAACTTCCAGGACATCATGCAAAAGCAAAGAC CAGAAAGAGTGTCTCATCTTCTTCAAGATAACTTGCCAAAATCTGTTTCCACTTTTCAGTATGATCGTTT CTTTGAGAAAAAAATTGATGAGAAAAAGAATGACCACACCTATCGAGTTTTTAAAACTGTGAACCGGCGA GCACACATCTTCCCCATGGCAGATGACTATTCAGACTCCCTCATCACCAAAAAGCAAGTGTCAGTCTGGT GCAGTAATGACTACCTAGGAATGAGTCGCCACCCACGGGTGTGTGGGGCAGTTATGGACACTTTGAAACA ACATGGTGCTGGGGCAGGTGGTACTAGAAATATTTCTGGAACTAGTAAATTCCATGTGGACTTAGAGCGG GAGCTGGCAGACCTCCATGGGAAAGATGCCGCACTCTTGTTTTCCTCGTGCTTTGTGGCCAATGACTCAA CCCTCTTCACCCTGGCTAAGATGATGCCAGGCTGTGAGATTTACTCTGATTCTGGGAACCATGCCTCCAT GATCCAAGGGATTCGAAACAGCCGAGTGCCAAAGTACATCTTCCGCCACAATGATGTCAGCCACCTCAGA GAACTGCTGCAAAGATCTGACCCCTCAGTCCCCAAGATTGTGGCATTTGAAACTGTCCATTCAATGGATG GGGCGGTGTGCCCACTGGAAGAGCTGTGTGATGTGGCCCATGAGTTTGGAGCAATCACCTTCGTGGATGA GGTCCACGCAGTGGGGCTTTATGGGGCTCGAGGCGGAGGGATTGGGGATCGGGATGGAGTCATGCCAAAA ATGGACATCATTTCTGGAACACTTGGCAAAGCCTTTGGTTGTGTTGGAGGGTACATCGCCAGCACGAGTT CTCTGATTGACACCGTACGGTCCTATGCTGCTGGCTTCATCTTCACCACCTCTCTGCCACCCATGCTGCT GGCTGGAGCCCTGGAGTCTGTGCGGATCCTGAAGAGCGCTGAGGGACGGGTGCTTCGCCGCCAGCACCAG CGCAACGTCAAACTCATGAGACAGATGCTAATGGATGCCGGCCTCCCTGTTGTCCACTGCCCCAGCCACA TCATCCCTGTGCGGGTTGCAGATGCTGCTAAAAACACAGAAGTCTGTGATGAACTAATGAGCAGACATAA CATCTACGTGCAAGCAATCAATTACCCTACGGTGCCCCGGGGAGAAGAGCTCCTACGGATTGCCCCCACC CCTCACCACACACCCCAGATGATGAACTACTTCCTTGAGAATCTGCTAGTCACATGGAAGCAAGTGGGGC TGGAACTGAAGCCTCATTCCTCAGCTGAGTGCAACTTCTGCAGGAGGCCACTGCATTTTGAAGTGATGAG TGAAAGAGAGAAGTCCTATTTCTCAGGCTTGAGCAAGTTGGTATCTGCTCAGGCCTGAGCATGACCTCAA TTATTTCACTTAACCCCAGGCCATTATCATATCCAGATGGTCTTCAGAGTTGTCTTTATATGTGAATTAA GTTATATTAAATTTTAATCTATAGTAAAAACATAGTCCTGGAAATAAATTCTTGCTTAAATGGTG [00181] NM_001304443.1 Homo sapiens 5'-aminolevulinate synthase 1 (ALAS1), transcript variant 3, mRNA; nuclear gene for mitochondrial product (SEQ ID NO: 2) CAGAAGAAGGCAGCGCCCAAGGCGCATGCGCAGCGGTCACTCCCGCTGTATATTAAGGCGCCGGCGATCG -57- 4892-3380-3674.1
Atty. Dkt. No.: 115872-2919 CGGCCTGAGGCTGCTCCCGGACAAGGGCAACGAGCGTTTCGTTTGGACTTCTCGACTTGAGTGCCCGCCT CCTTCGCCGCCGCCTCTGCAGTCCTCAGCGCAGGAGCCAGCATACTTCCTGAACATGGAGAGTGTTGTTC GCCGCTGCCCATTCTTATCCCGAGTCCCCCAGGCCTTTCTGCAGAAAGCAGGCAAATCTCTGTTGTTCTA TGCCCAAAACTGCCCCAAGATGATGGAAGTTGGGGCCAAGCCAGCCCCTCGGGCATTGTCCACTGCAGCA GTACACTACCAACAGATCAAAGAAACCCCTCCGGCCAGTGAGAAAGACAAAACTGCTAAGGCCAAGGTCC AACAGACTCCTGATGGATCCCAGCAGAGTCCAGATGGCACACAGCTTCCGTCTGGACACCCCTTGCCTGC CACAAGCCAGGGCACTGCAAGCAAATGCCCTTTCCTGGCAGCACAGATGAATCAGAGAGGCAGCAGTGTC TTCTGCAAAGCCAGTCTTGAGCTTCAGGAGGATGTGCAGGAAATGAATGCCGTGAGGAAAGAGGTTGCTG AAACCTCAGCAGGCCCCAGTGTGGTTAGTGTGAAAACCGATGGAGGGGATCCCAGTGGACTGCTGAAGAA CTTCCAGGACATCATGCAAAAGCAAAGACCAGAAAGAGTGTCTCATCTTCTTCAAGATAACTTGCCAAAA TCTGTTTCCACTTTTCAGTATGATCGTTTCTTTGAGAAAAAAATTGATGAGAAAAAGAATGACCACACCT ATCGAGTTTTTAAAACTGTGAACCGGCGAGCACACATCTTCCCCATGGCAGATGACTATTCAGACTCCCT CATCACCAAAAAGCAAGTGTCAGTCTGGTGCAGTAATGACTACCTAGGAATGAGTCGCCACCCACGGGTG TGTGGGGCAGTTATGGACACTTTGAAACAACATGGTGCTGGGGCAGGTGGTACTAGAAATATTTCTGGAA CTAGTAAATTCCATGTGGACTTAGAGCGGGAGCTGGCAGACCTCCATGGGAAAGATGCCGCACTCTTGTT TTCCTCGTGCTTTGTGGCCAATGACTCAACCCTCTTCACCCTGGCTAAGATGATGCCAGGCTGTGAGATT TACTCTGATTCTGGGAACCATGCCTCCATGATCCAAGGGATTCGAAACAGCCGAGTGCCAAAGTACATCT TCCGCCACAATGATGTCAGCCACCTCAGAGAACTGCTGCAAAGATCTGACCCCTCAGTCCCCAAGATTGT GGCATTTGAAACTGTCCATTCAATGGATGGGGCGGTGTGCCCACTGGAAGAGCTGTGTGATGTGGCCCAT GAGTTTGGAGCAATCACCTTCGTGGATGAGGTCCACGCAGTGGGGCTTTATGGGGCTCGAGGCGGAGGGA TTGGGGATCGGGATGGAGTCATGCCAAAAATGGACATCATTTCTGGAACACTTGGCAAAGCCTTTGGTTG TGTTGGAGGGTACATCGCCAGCACGAGTTCTCTGATTGACACCGTACGGTCCTATGCTGCTGGCTTCATC TTCACCACCTCTCTGCCACCCATGCTGCTGGCTGGAGCCCTGGAGTCTGTGCGGATCCTGAAGAGCGCTG AGGGACGGGTGCTTCGCCGCCAGCACCAGCGCAACGTCAAACTCATGAGACAGATGCTAATGGATGCCGG CCTCCCTGTTGTCCACTGCCCCAGCCACATCATCCCTGTGCGGGTTGCAGATGCTGCTAAAAACACAGAA GTCTGTGATGAACTAATGAGCAGACATAACATCTACGTGCAAGCAATCAATTACCCTACGGTGCCCCGGG GAGAAGAGCTCCTACGGATTGCCCCCACCCCTCACCACACACCCCAGATGATGAACTACTTCCTTGAGAA TCTGCTAGTCACATGGAAGCAAGTGGGGCTGGAACTGAAGCCTCATTCCTCAGCTGAGTGCAACTTCTGC AGGAGGCCACTGCATTTTGAAGTGATGAGTGAAAGAGAGAAGTCCTATTTCTCAGGCTTGAGCAAGTTGG TATCTGCTCAGGCCTGAGCATGACCTCAATTATTTCACTTAACCCCAGGCCATTATCATATCCAGATGGT CTTCAGAGTTGTCTTTATATGTGAATTAAGTTATATTAAATTTTAATCTATAGTAAAAACATAGTCCTGG AAATAAATTCTTGCTTAAATGGTGAAAAAA [00182] NM_001304444.1 Homo sapiens 5'-aminolevulinate synthase 1 (ALAS1), transcript variant 4, mRNA; nuclear gene for mitochondrial product (SEQ ID NO: 3) CAGAAGAAGGCAGCGCCCAAGGCGCATGCGCAGCGGTCACTCCCGCTGTATATTAAGGCGCCGGCGATCG CGGCCTGAGGCTGCTCCCGGACAAGGGCAACGAGCGTTTCGTTTGGACTTCTCGACTTGAGTGCCCGCCT CCTTCGCCGCCGCCTCTGCAGTCCTCAGCGCAGTTATGCCCAGTTCTTCCCGCTGTGGGGACACGACCAC GGAGGAATCCTTGCTTCAGGGACTCGGGACCCTGCTGGACCCCTTCCTCGGGTTTAGGGGATGTGGGGAC CAGGAGAAAGTCAGGATCCCTAAGAGTCTTCCCTGCCTGGATGGATGAGTGGCTTCTTCTCCACCTAGAT GAGCCAGCATACTTCCTGAACATGGAGAGTGTTGTTCGCCGCTGCCCATTCTTATCCCGAGTCCCCCAGG CCTTTCTGCAGAAAGCAGGCAAATCTCTGTTGTTCTATGCCCAAAACTGCCCCAAGATGATGGAAGTTGG GGCCAAGCCAGCCCCTCGGGCATTGTCCACTGCAGCAGTACACTACCAACAGATCAAAGAAACCCCTCCG GCCAGTGAGAAAGACAAAACTGCTAAGGCCAAGGTCCAACAGACTCCTGATGGATCCCAGCAGAGTCCAG ATGGCACACAGCTTCCGTCTGGACACCCCTTGCCTGCCACAAGCCAGGGCACTGCAAGCAAATGCCCTTT CCTGGCAGCACAGATGAATCAGAGAGGCAGCAGTGTCTTCTGCAAAGCCAGTCTTGAGCTTCAGGAGGAT GTGCAGGAAATGAATGCCGTGAGGAAAGAGGTTGCTGAAACCTCAGCAGGCCCCAGTGTGGTTAGTGTGA AAACCGATGGAGGGGATCCCAGTGGACTGCTGAAGAACTTCCAGGACATCATGCAAAAGCAAAGACCAGA AAGAGTGTCTCATCTTCTTCAAGATAACTTGCCAAAATCTGTTTCCACTTTTCAGTATGATCGTTTCTTT GAGAAAAAAATTGATGAGAAAAAGAATGACCACACCTATCGAGTTTTTAAAACTGTGAACCGGCGAGCAC ACATCTTCCCCATGGCAGATGACTATTCAGACTCCCTCATCACCAAAAAGCAAGTGTCAGTCTGGTGCAG -58- 4892-3380-3674.1
Atty. Dkt. No.: 115872-2919 TAATGACTACCTAGGAATGAGTCGCCACCCACGGGTGTGTGGGGCAGTTATGGACACTTTGAAACAACAT GGTGCTGGGGCAGGTGGTACTAGAAATATTTCTGGAACTAGTAAATTCCATGTGGACTTAGAGCGGGAGC TGGCAGACCTCCATGGGAAAGATGCCGCACTCTTGTTTTCCTCGTGCTTTGTGGCCAATGACTCAACCCT CTTCACCCTGGCTAAGATGATGCCAGGCTGTGAGATTTACTCTGATTCTGGGAACCATGCCTCCATGATC CAAGGGATTCGAAACAGCCGAGTGCCAAAGTACATCTTCCGCCACAATGATGTCAGCCACCTCAGAGAAC TGCTGCAAAGATCTGACCCCTCAGTCCCCAAGATTGTGGCATTTGAAACTGTCCATTCAATGGATGGGGC GGTGTGCCCACTGGAAGAGCTGTGTGATGTGGCCCATGAGTTTGGAGCAATCACCTTCGTGGATGAGGTC CACGCAGTGGGGCTTTATGGGGCTCGAGGCGGAGGGATTGGGGATCGGGATGGAGTCATGCCAAAAATGG ACATCATTTCTGGAACACTTGGCAAAGCCTTTGGTTGTGTTGGAGGGTACATCGCCAGCACGAGTTCTCT GATTGACACCGTACGGTCCTATGCTGCTGGCTTCATCTTCACCACCTCTCTGCCACCCATGCTGCTGGCT GGAGCCCTGGAGTCTGTGCGGATCCTGAAGAGCGCTGAGGGACGGGTGCTTCGCCGCCAGCACCAGCGCA ACGTCAAACTCATGAGACAGATGCTAATGGATGCCGGCCTCCCTGTTGTCCACTGCCCCAGCCACATCAT CCCTGTGCGGGTTGCAGATGCTGCTAAAAACACAGAAGTCTGTGATGAACTAATGAGCAGACATAACATC TACGTGCAAGCAATCAATTACCCTACGGTGCCCCGGGGAGAAGAGCTCCTACGGATTGCCCCCACCCCTC ACCACACACCCCAGATGATGAACTACTTCCTTGAGAATCTGCTAGTCACATGGAAGCAAGTGGGGCTGGA ACTGAAGCCTCATTCCTCAGCTGAGTGCAACTTCTGCAGGAGGCCACTGCATTTTGAAGTGATGAGTGAA AGAGAGAAGTCCTATTTCTCAGGCTTGAGCAAGTTGGTATCTGCTCAGGCCTGAGCATGACCTCAATTAT TTCACTTAACCCCAGGCCATTATCATATCCAGATGGTCTTCAGAGTTGTCTTTATATGTGAATTAAGTTA TATTAAATTTTAATCTATAGTAAAAACATAGTCCTGGAAATAAATTCTTGCTTAAATGGTGAAAAAA [00183] NM_199166.2 Homo sapiens 5'-aminolevulinate synthase 1 (ALAS1), transcript variant 2, mRNA; nuclear gene for mitochondrial product (SEQ ID NO: 4) CAGAAGAAGGCAGCGCCCAAGGCGCATGCGCAGCGGTCACTCCCGCTGTATATTAAGGCGCCGGCGATCG CGGCCTGAGGCTGCTCCCGGACAAGGGCAACGAGCGTTTCGTTTGGACTTCTCGACTTGAGTGCCCGCCT CCTTCGCCGCCGCCTCTGCAGTCCTCAGCGCAGTCTTTCCACAGGAGCCAGCATACTTCCTGAACATGGA GAGTGTTGTTCGCCGCTGCCCATTCTTATCCCGAGTCCCCCAGGCCTTTCTGCAGAAAGCAGGCAAATCT CTGTTGTTCTATGCCCAAAACTGCCCCAAGATGATGGAAGTTGGGGCCAAGCCAGCCCCTCGGGCATTGT CCACTGCAGCAGTACACTACCAACAGATCAAAGAAACCCCTCCGGCCAGTGAGAAAGACAAAACTGCTAA GGCCAAGGTCCAACAGACTCCTGATGGATCCCAGCAGAGTCCAGATGGCACACAGCTTCCGTCTGGACAC CCCTTGCCTGCCACAAGCCAGGGCACTGCAAGCAAATGCCCTTTCCTGGCAGCACAGATGAATCAGAGAG GCAGCAGTGTCTTCTGCAAAGCCAGTCTTGAGCTTCAGGAGGATGTGCAGGAAATGAATGCCGTGAGGAA AGAGGTTGCTGAAACCTCAGCAGGCCCCAGTGTGGTTAGTGTGAAAACCGATGGAGGGGATCCCAGTGGA CTGCTGAAGAACTTCCAGGACATCATGCAAAAGCAAAGACCAGAAAGAGTGTCTCATCTTCTTCAAGATA ACTTGCCAAAATCTGTTTCCACTTTTCAGTATGATCGTTTCTTTGAGAAAAAAATTGATGAGAAAAAGAA TGACCACACCTATCGAGTTTTTAAAACTGTGAACCGGCGAGCACACATCTTCCCCATGGCAGATGACTAT TCAGACTCCCTCATCACCAAAAAGCAAGTGTCAGTCTGGTGCAGTAATGACTACCTAGGAATGAGTCGCC ACCCACGGGTGTGTGGGGCAGTTATGGACACTTTGAAACAACATGGTGCTGGGGCAGGTGGTACTAGAAA TATTTCTGGAACTAGTAAATTCCATGTGGACTTAGAGCGGGAGCTGGCAGACCTCCATGGGAAAGATGCC GCACTCTTGTTTTCCTCGTGCTTTGTGGCCAATGACTCAACCCTCTTCACCCTGGCTAAGATGATGCCAG GCTGTGAGATTTACTCTGATTCTGGGAACCATGCCTCCATGATCCAAGGGATTCGAAACAGCCGAGTGCC AAAGTACATCTTCCGCCACAATGATGTCAGCCACCTCAGAGAACTGCTGCAAAGATCTGACCCCTCAGTC CCCAAGATTGTGGCATTTGAAACTGTCCATTCAATGGATGGGGCGGTGTGCCCACTGGAAGAGCTGTGTG ATGTGGCCCATGAGTTTGGAGCAATCACCTTCGTGGATGAGGTCCACGCAGTGGGGCTTTATGGGGCTCG AGGCGGAGGGATTGGGGATCGGGATGGAGTCATGCCAAAAATGGACATCATTTCTGGAACACTTGGCAAA GCCTTTGGTTGTGTTGGAGGGTACATCGCCAGCACGAGTTCTCTGATTGACACCGTACGGTCCTATGCTG CTGGCTTCATCTTCACCACCTCTCTGCCACCCATGCTGCTGGCTGGAGCCCTGGAGTCTGTGCGGATCCT GAAGAGCGCTGAGGGACGGGTGCTTCGCCGCCAGCACCAGCGCAACGTCAAACTCATGAGACAGATGCTA ATGGATGCCGGCCTCCCTGTTGTCCACTGCCCCAGCCACATCATCCCTGTGCGGGTTGCAGATGCTGCTA AAAACACAGAAGTCTGTGATGAACTAATGAGCAGACATAACATCTACGTGCAAGCAATCAATTACCCTAC -59- 4892-3380-3674.1
Atty. Dkt. No.: 115872-2919 GGTGCCCCGGGGAGAAGAGCTCCTACGGATTGCCCCCACCCCTCACCACACACCCCAGATGATGAACTAC TTCCTTGAGAATCTGCTAGTCACATGGAAGCAAGTGGGGCTGGAACTGAAGCCTCATTCCTCAGCTGAGT GCAACTTCTGCAGGAGGCCACTGCATTTTGAAGTGATGAGTGAAAGAGAGAAGTCCTATTTCTCAGGCTT GAGCAAGTTGGTATCTGCTCAGGCCTGAGCATGACCTCAATTATTTCACTTAACCCCAGGCCATTATCAT ATCCAGATGGTCTTCAGAGTTGTCTTTATATGTGAATTAAGTTATATTAAATTTTAATCTATAGTAAAAA CATAGTCCTGGAAATAAATTCTTGCTTAAATGGTGAAAAAA [00184] NM_000032.5 Homo sapiens 5'-aminolevulinate synthase 2 (ALAS2), transcript variant 1, mRNA; nuclear gene for mitochondrial product (SEQ ID NO: 5) ACCTGTCATTCGTTCGTCCTCAGTGCAGGGCAACAGGACTTTAGGTTCAAGATGGTGACTGCAGCCATGC TGCTACAGTGCTGCCCAGTGCTTGCCCGGGGCCCCACAAGCCTCCTAGGCAAGGTGGTTAAGACTCACCA GTTCCTGTTTGGTATTGGACGCTGTCCCATCCTGGCTACCCAAGGACCAAACTGTTCTCAAATCCACCTT AAGGCAACAAAGGCTGGAGGAGATTCTCCATCTTGGGCGAAGGGCCACTGTCCCTTCATGCTGTCGGAAC TCCAGGATGGGAAGAGCAAGATTGTGCAGAAGGCAGCCCCAGAAGTCCAGGAAGATGTGAAGGCTTTCAA GACAGATCTGCCTAGCTCCCTGGTCTCAGTCAGCCTAAGGAAGCCATTTTCCGGTCCCCAGGAGCAGGAG CAGATCTCTGGGAAGGTCACACACCTGATTCAGAACAATATGCCTGGAAACTATGTCTTCAGTTATGACC AGTTTTTCAGGGACAAGATCATGGAGAAGAAACAGGATCACACCTACCGTGTGTTCAAGACTGTGAACCG CTGGGCTGATGCATATCCCTTTGCCCAACATTTCTCTGAGGCATCTGTGGCCTCAAAGGATGTGTCCGTC TGGTGTAGTAATGATTACCTGGGCATGAGCCGACACCCTCAGGTCTTGCAAGCCACACAGGAGACCCTGC AGCGTCATGGTGCTGGAGCTGGTGGCACCCGCAACATCTCAGGCACCAGTAAGTTTCATGTGGAGCTTGA GCAGGAGCTGGCTGAGCTGCACCAGAAGGACTCAGCCCTGCTCTTCTCCTCCTGCTTTGTTGCCAATGAC TCTACTCTCTTCACCTTGGCCAAGATCCTGCCAGGGTGCGAGATTTACTCAGACGCAGGCAACCATGCTT CCATGATCCAAGGTATCCGTAACAGTGGAGCAGCCAAGTTTGTCTTCAGGCACAATGACCCTGACCACCT AAAGAAACTTCTAGAGAAGTCTAACCCTAAGATACCCAAAATTGTGGCCTTTGAGACTGTCCACTCCATG GATGGTGCCATCTGTCCCCTCGAGGAGTTGTGTGATGTGTCCCACCAGTATGGGGCCCTGACCTTCGTGG ATGAGGTCCATGCTGTAGGACTGTATGGGTCCCGGGGCGCTGGGATTGGGGAGCGTGATGGAATTATGCA TAAGATTGACATCATCTCTGGAACTCTTGGCAAGGCCTTTGGCTGTGTGGGCGGCTACATTGCCAGCACC CGTGACTTGGTGGACATGGTGCGCTCCTATGCTGCAGGCTTCATCTTTACCACTTCTCTGCCCCCCATGG TGCTCTCTGGAGCTCTAGAATCTGTGCGGCTGCTCAAGGGAGAGGAGGGCCAAGCCCTGAGGCGAGCCCA CCAGCGCAATGTCAAGCACATGCGCCAGCTACTCATGGACAGGGGCCTTCCTGTCATCCCCTGCCCCAGC CACATCATCCCCATCCGGGTGGGCAATGCAGCACTCAACAGCAAGCTCTGTGATCTCCTGCTCTCCAAGC ATGGCATCTATGTGCAGGCCATCAACTACCCAACTGTCCCCCGGGGTGAAGAGCTCCTGCGCTTGGCACC CTCCCCCCACCACAGCCCTCAGATGATGGAAGATTTTGTGGAGAAGCTGCTGCTGGCTTGGACTGCGGTG GGGCTGCCCCTCCAGGATGTGTCTGTGGCTGCCTGCAATTTCTGTCGCCGTCCTGTACACTTTGAGCTCA TGAGTGAGTGGGAACGTTCCTACTTCGGGAACATGGGGCCCCAGTATGTCACCACCTATGCCTGAGAAGC CAGCTGCCTAGGATTCACACCCCACCTGCGCTTCACTTGGGTCCAGGCCTACTCCTGTCTTCTGCTTTGT TGTGTGCCTCTAGCTGAATTGAGCCTAAAAATAAAGCACAAACCACAGCA [00185] NM_001037967.4 Homo sapiens 5'-aminolevulinate synthase 2 (ALAS2), transcript variant 2, mRNA; nuclear gene for mitochondrial product (SEQ ID NO: 6) ACCTGTCATTCGTTCGTCCTCAGTGCAGGGCAACAGGACTTTAGGTTCAAGATGGTGACTGCAGCCATGC TGCTACAGTGCTGCCCAGTGCTTGCCCGGGGCCCCACAAGCCTCCTAGGCAAGGTGGTTAAGACTCACCA GTTCCTGTTTGGTATTGGACGCTGTCCCATCCTGGCTACCCAAGGACCAAACTGTTCTCAAATCCACCTT AAGGCAACAAAGGCTGGAGGAGATTCTCCATCTTGGGCGAAGGGCCACTGTCCCTTCATGCTGTCGGAAC TCCAGGATGGGAAGAGCAAGATTGTGCAGAAGGCAGCCCCAGAAGTCCAGGAAGATGTGAAGGCTTTCAA GACAGGAAACTATGTCTTCAGTTATGACCAGTTTTTCAGGGACAAGATCATGGAGAAGAAACAGGATCAC ACCTACCGTGTGTTCAAGACTGTGAACCGCTGGGCTGATGCATATCCCTTTGCCCAACATTTCTCTGAGG -60- 4892-3380-3674.1
Atty. Dkt. No.: 115872-2919 CATCTGTGGCCTCAAAGGATGTGTCCGTCTGGTGTAGTAATGATTACCTGGGCATGAGCCGACACCCTCA GGTCTTGCAAGCCACACAGGAGACCCTGCAGCGTCATGGTGCTGGAGCTGGTGGCACCCGCAACATCTCA GGCACCAGTAAGTTTCATGTGGAGCTTGAGCAGGAGCTGGCTGAGCTGCACCAGAAGGACTCAGCCCTGC TCTTCTCCTCCTGCTTTGTTGCCAATGACTCTACTCTCTTCACCTTGGCCAAGATCCTGCCAGGGTGCGA GATTTACTCAGACGCAGGCAACCATGCTTCCATGATCCAAGGTATCCGTAACAGTGGAGCAGCCAAGTTT GTCTTCAGGCACAATGACCCTGACCACCTAAAGAAACTTCTAGAGAAGTCTAACCCTAAGATACCCAAAA TTGTGGCCTTTGAGACTGTCCACTCCATGGATGGTGCCATCTGTCCCCTCGAGGAGTTGTGTGATGTGTC CCACCAGTATGGGGCCCTGACCTTCGTGGATGAGGTCCATGCTGTAGGACTGTATGGGTCCCGGGGCGCT GGGATTGGGGAGCGTGATGGAATTATGCATAAGATTGACATCATCTCTGGAACTCTTGGCAAGGCCTTTG GCTGTGTGGGCGGCTACATTGCCAGCACCCGTGACTTGGTGGACATGGTGCGCTCCTATGCTGCAGGCTT CATCTTTACCACTTCTCTGCCCCCCATGGTGCTCTCTGGAGCTCTAGAATCTGTGCGGCTGCTCAAGGGA GAGGAGGGCCAAGCCCTGAGGCGAGCCCACCAGCGCAATGTCAAGCACATGCGCCAGCTACTCATGGACA GGGGCCTTCCTGTCATCCCCTGCCCCAGCCACATCATCCCCATCCGGGTGGGCAATGCAGCACTCAACAG CAAGCTCTGTGATCTCCTGCTCTCCAAGCATGGCATCTATGTGCAGGCCATCAACTACCCAACTGTCCCC CGGGGTGAAGAGCTCCTGCGCTTGGCACCCTCCCCCCACCACAGCCCTCAGATGATGGAAGATTTTGTGG AGAAGCTGCTGCTGGCTTGGACTGCGGTGGGGCTGCCCCTCCAGGATGTGTCTGTGGCTGCCTGCAATTT CTGTCGCCGTCCTGTACACTTTGAGCTCATGAGTGAGTGGGAACGTTCCTACTTCGGGAACATGGGGCCC CAGTATGTCACCACCTATGCCTGAGAAGCCAGCTGCCTAGGATTCACACCCCACCTGCGCTTCACTTGGG TCCAGGCCTACTCCTGTCTTCTGCTTTGTTGTGTGCCTCTAGCTGAATTGAGCCTAAAAATAAAGCACAA ACCACAGCA [00186] NM_001037968.4 Homo sapiens 5'-aminolevulinate synthase 2 (ALAS2), transcript variant 3, mRNA; nuclear gene for mitochondrial product (SEQ ID NO: 7) ACCTGTCATTCGTTCGTCCTCAGTGCAGGGCAACAGGGCCCACATGCCTTGGCCCCACATACCAACCCAG GCTGCTGTGACAGCCCATGAGAGGGGGAGAGGTTGCTCTGGGATGGAACAAGAAAAAGAGGTTGTTTTGT GAGGACTTTAGGTTCAAGATGGTGACTGCAGCCATGCTGCTACAGTGCTGCCCAGTGCTTGCCCGGGGCC CCACAAGCCTCCTAGGCAAGGTGGTTAAGACTCACCAGTTCCTGTTTGGTATTGGACGCTGTCCCATCCT GGCTACCCAAGGACCAAACTGTTCTCAAATCCACCTTAAGGCAACAAAGGCTGGAGGAGATTCTCCATCT TGGGCGAAGGGCCACTGTCCCTTCATGCTGTCGGAACTCCAGGATGGGAAGAGCAAGATTGTGCAGAAGG CAGCCCCAGAAGTCCAGGAAGATGTGAAGGCTTTCAAGACAGGAAACTATGTCTTCAGTTATGACCAGTT TTTCAGGGACAAGATCATGGAGAAGAAACAGGATCACACCTACCGTGTGTTCAAGACTGTGAACCGCTGG GCTGATGCATATCCCTTTGCCCAACATTTCTCTGAGGCATCTGTGGCCTCAAAGGATGTGTCCGTCTGGT GTAGTAATGATTACCTGGGCATGAGCCGACACCCTCAGGTCTTGCAAGCCACACAGGAGACCCTGCAGCG TCATGGTGCTGGAGCTGGTGGCACCCGCAACATCTCAGGCACCAGTAAGTTTCATGTGGAGCTTGAGCAG GAGCTGGCTGAGCTGCACCAGAAGGACTCAGCCCTGCTCTTCTCCTCCTGCTTTGTTGCCAATGACTCTA CTCTCTTCACCTTGGCCAAGATCCTGCCAGGGTGCGAGATTTACTCAGACGCAGGCAACCATGCTTCCAT GATCCAAGGTATCCGTAACAGTGGAGCAGCCAAGTTTGTCTTCAGGCACAATGACCCTGACCACCTAAAG AAACTTCTAGAGAAGTCTAACCCTAAGATACCCAAAATTGTGGCCTTTGAGACTGTCCACTCCATGGATG GTGCCATCTGTCCCCTCGAGGAGTTGTGTGATGTGTCCCACCAGTATGGGGCCCTGACCTTCGTGGATGA GGTCCATGCTGTAGGACTGTATGGGTCCCGGGGCGCTGGGATTGGGGAGCGTGATGGAATTATGCATAAG ATTGACATCATCTCTGGAACTCTTGGCAAGGCCTTTGGCTGTGTGGGCGGCTACATTGCCAGCACCCGTG ACTTGGTGGACATGGTGCGCTCCTATGCTGCAGGCTTCATCTTTACCACTTCTCTGCCCCCCATGGTGCT CTCTGGAGCTCTAGAATCTGTGCGGCTGCTCAAGGGAGAGGAGGGCCAAGCCCTGAGGCGAGCCCACCAG CGCAATGTCAAGCACATGCGCCAGCTACTCATGGACAGGGGCCTTCCTGTCATCCCCTGCCCCAGCCACA TCATCCCCATCCGGGTGGGCAATGCAGCACTCAACAGCAAGCTCTGTGATCTCCTGCTCTCCAAGCATGG CATCTATGTGCAGGCCATCAACTACCCAACTGTCCCCCGGGGTGAAGAGCTCCTGCGCTTGGCACCCTCC CCCCACCACAGCCCTCAGATGATGGAAGATTTTGTGGAGAAGCTGCTGCTGGCTTGGACTGCGGTGGGGC TGCCCCTCCAGGATGTGTCTGTGGCTGCCTGCAATTTCTGTCGCCGTCCTGTACACTTTGAGCTCATGAG TGAGTGGGAACGTTCCTACTTCGGGAACATGGGGCCCCAGTATGTCACCACCTATGCCTGAGAAGCCAGC TGCCTAGGATTCACACCCCACCTGCGCTTCACTTGGGTCCAGGCCTACTCCTGTCTTCTGCTTTGTTGTG TGCCTCTAGCTGAATTGAGCCTAAAAATAAAGCACAAACCACAGCA -61- 4892-3380-3674.1
Atty. Dkt. No.: 115872-2919 [00187] Antisense Nucleic Acids. In one aspect, the present disclosure provides ALAS1/ALAS2-specific inhibitory nucleic acids comprising a nucleic acid molecule which is complementary to a portion of an ALAS1/ALAS2 nucleic acid sequence selected from the group consisting of SEQ ID NOs: 1-7. [00188] The present disclosure also provides an antisense nucleic acid comprising a nucleic acid sequence that is complementary to and specifically hybridizes with a portion of any one of SEQ ID NOs: 1-7 (ALAS1/ALAS2 mRNA), thereby reducing or inhibiting ALAS1/ALAS2 expression. The antisense nucleic acid may be antisense RNA, or antisense DNA. Antisense nucleic acids based on the known ALAS1/ALAS2 gene sequence can be readily designed and engineered using methods known in the art. In some embodiments, the antisense nucleic acid comprises the nucleic acid sequence of SEQ ID NO: 8, SEQ ID NO: 9, SEQ ID NO: 10, SEQ ID NO: 11, SEQ ID NO: 95, SEQ ID NO: 96, or a complement thereof. [00189] Antisense nucleic acids are molecules which are complementary to a sense nucleic acid strand, e.g., complementary to the coding strand of a double-stranded DNA molecule (or cDNA) or complementary to an mRNA sequence. Accordingly, an antisense nucleic acid can form hydrogen bonds with a sense nucleic acid. The antisense nucleic acid can be complementary to an entire ALAS1/ALAS2 coding strand, or to a portion thereof, e.g., all or part of the protein coding region (or open reading frame). In some embodiments, the antisense nucleic acid is an oligonucleotide which is complementary to only a portion of the coding region of ALAS1/ALAS2 mRNA. In certain embodiments, an antisense nucleic acid molecule can be complementary to a noncoding region of the ALAS1/ALAS2 coding strand. In some embodiments, the noncoding region refers to the 5′ and 3′ untranslated regions that flank the coding region and are not translated into amino acids. For example, the antisense oligonucleotide can be complementary to the region surrounding the translation start site of ALAS1/ALAS2. An antisense oligonucleotide can be, for example, about 5, 10, 15, 20, 25, 30, 35, 40, 45 or 50 nucleotides in length. [00190] An antisense nucleic acid can be constructed using chemical synthesis and enzymatic ligation reactions using procedures known in the art. For example, an antisense nucleic acid -62- 4892-3380-3674.1
Atty. Dkt. No.: 115872-2919 (e.g., an antisense oligonucleotide) can be chemically synthesized using naturally occurring nucleotides or modified nucleotides designed to increase the biological stability of the molecules or to increase the physical stability of the duplex formed between the antisense and sense nucleic acids, e.g., phosphorothioate derivatives and acridine substituted nucleotides. Examples of modified nucleotides which can be used to generate the antisense nucleic acid include 5- fluorouracil, 5-bromouracil, 5-chlorouracil, 5-hodouracil, hypoxanthine, xanthine, 4- acetylcytosine, 5-(carboxyhydroxylmethyl)uracil, 5-carboxymethylaminomethyl-2-thouridine, 5- carboxymethylaminometh-yluracil, dihydrouracil, beta-D-galactosylqueosine, inosine, N6- isopentenyladenine, 1-methylguanine, 1-methylinosine, 2,2-dimethylguanine, 2-methyladenine, 2-methylguanine, 3-methylcytosine, 5-metnylcytosine, N6-adenine, 7-methylguanine, 5- methylaminomethyluracil, 5-methoxyaminomethyl-2-thiouracil, beta-D-mannosylqueosine, 5′- methoxycarboxymethyluracil, 5-methoxyuracil, 2-methylthio-N6-isopenten-yladenine, uracil-5- oxyacetic acid (v), wybutosine, pseudouracil, queosine, 2-thiocytosine, 5-methyl-2-thiouracil, 2- thlouracil, 4-thiouracil, 5-methyluracil, uracil-5-oxyacetic acid methylester, uracil-5-cxyacetic acid (v), 5-methyl-2-thiouracil, 3-(3-amino-3-N-2-carboxypropyl) uracil, (acp3)w, and 2,6- diaminopurine. Alternatively, the antisense nucleic acid can be produced biologically using an expression vector into which a nucleic acid has been subcloned in an antisense orientation (i.e., RNA transcribed from the inserted nucleic acid will be of an antisense orientation to a target nucleic acid of interest). [00191] The antisense nucleic acid molecules may be administered to a subject or generated in situ such that they hybridize with or bind to cellular mRNA and/or genomic DNA encoding the protein of interest to thereby inhibit expression of the protein, e.g., by inhibiting transcription and/or translation. The hybridization can occur via Watson-Crick base pairing to form a stable duplex, or in the case of an antisense nucleic acid molecule which binds to DNA duplexes, through specific interactions in the major groove of the double helix. [00192] In some embodiments, the antisense nucleic acid molecules are modified such that they specifically bind to receptors or antigens expressed on a selected cell surface, e.g., by linking the antisense nucleic acid molecules to peptides or antibodies which bind to cell surface -63- 4892-3380-3674.1
Atty. Dkt. No.: 115872-2919 receptors or antigens. In some embodiments, the antisense nucleic acid molecule is an alpha- anomeric nucleic acid molecule. An alpha-anomeric nucleic acid molecule forms specific double-stranded hybrids with complementary RNA in which, contrary to the usual β-units, the strands run parallel to each other (Gaultier et al., Nucleic Acids. Res.15:6625-6641(1987)). The antisense nucleic acid molecule can also comprise a 2′-O -methylribonucleotide (Inoue et al., Nucleic Acids Res.15:6131-6148 (1987)) or a chimeric RNA-DNA analogue (Inoue et al., FEBS Lett.215:327-330 (1987)). [00193] siRNAs. The present disclosure also provides a short hairpin RNA (shRNA) or small interfering RNA (siRNA) comprising a nucleic acid sequence that is complementary to and specifically hybridizes with a portion of any one of SEQ ID NOs: 1-7 (ALAS1/ALAS2 mRNA), thereby reducing or inhibiting ALAS1/ALAS2 expression. In some embodiments, the shRNA or siRNA is about 18, 19, 20, 21, 22, 23, 24, 25, 26, 27, 28 or 29 base pairs in length. Double- stranded RNA (dsRNA) can induce sequence-specific post-transcriptional gene silencing (e.g., RNA interference (RNAi)) in many organisms such as C. elegans, Drosophila, plants, mammals, oocytes and early embryos. RNAi is a process that interferes with or significantly reduces the number of protein copies made by an mRNA. For example, a double-stranded siRNA or shRNA molecule is engineered to complement and hydridize to a mRNA of a target gene. Following intracellular delivery, the siRNA or shRNA molecule associates with an RNA-induced silencing complex (RISC), which then binds and degrades a complementary target mRNA (such as ALAS1/ALAS2 mRNA). In some embodiments, the shRNA or siRNA comprises the nucleic acid sequence of SEQ ID NO: 8, SEQ ID NO: 9, SEQ ID NO: 10, SEQ ID NO: 11, SEQ ID NO: 95, or SEQ ID NO: 96. [00194] Ribozymes. The present disclosure also provides a ribozyme comprising a nucleic acid sequence that is complementary to and specifically hybridizes with a portion of any one of SEQ ID NOs: 1-7 (ALAS1/ALAS2 mRNA), thereby reducing or inhibiting ALAS1/ALAS2 expression. Ribozymes are catalytic RNA molecules with ribonuclease activity which are capable of cleaving a complementary single-stranded nucleic acid, such as an mRNA. Thus, ribozymes (e.g., hammerhead ribozymes (described in Haselhoff and Gerlach, Nature 334:585- -64- 4892-3380-3674.1
Atty. Dkt. No.: 115872-2919 591 (1988))) can be used to catalytically cleave ALAS1/ALAS2 transcripts, thereby inhibiting translation of ALAS1/ALAS2. [00195] A ribozyme having specificity for an ALAS1/ALAS2-encoding nucleic acid can be designed based upon an ALAS1/ALAS2 nucleic acid sequence disclosed herein. For example, a derivative of a Tetrahymena L-19 IVS RNA can be constructed in which the nucleotide sequence of the active site is complementary to the nucleotide sequence to be cleaved in an ALAS1/ALAS2- encoding mRNA. See, e.g., U.S. Pat. No.4,987,071 and U.S. Pat. No.5,116,742. Alternatively, ALAS1/ALAS2 mRNA can be used to select a catalytic RNA having a specific ribonuclease activity from a pool of RNA molecules. See, e.g., Bartel and Szostak (1993) Science 261:1411- 1418, incorporated herein by reference. [00196] sqRNAs. The present disclosure also provides a synthetic guide RNA (sgRNA) comprising a nucleic acid sequence that is complementary to and specifically hybridizes with a portion of any one of SEQ ID NOs: 1-7 (ALAS1/ALAS2 mRNA). Guide RNAs for use in CRISPR-Cas systems are typically generated as a single guide RNA comprising a crRNA segment and a tracrRNA segment. The crRNA segment and a tracrRNA segment can also be generated as separate RNA molecules. The crRNA segment comprises the targeting sequence that binds to a portion of any one of SEQ ID NOs: 1-7, and a stem portion that hybridizes to a tracrRNA. The tracrRNA segment comprises a nucleotide sequence that is partially or completely complementary to the stem sequence of the crRNA and a nucleotide sequence that binds to the CRISPR enzyme. In some embodiments, the crRNA segment and the tracrRNA segment are provided as a single guide RNA. In some embodiments, the crRNA segment and the tracrRNA segment are provided as separate RNAs. The combination of the CRISPR enzyme with the crRNA and tracrRNA make up a functional CRISPR-Cas system. Exemplary CRISPR- Cas systems for targeting nucleic acids, are described, for example, in WO2015/089465. [00197] In some embodiments, a synthetic guide RNA is a single RNA represented as comprising the following elements: 5ʹ-X1-X2-Y-Z-3ʹ -65- 4892-3380-3674.1
Atty. Dkt. No.: 115872-2919 [00198] where X1 and X2 represent the crRNA segment, where X1 is the targeting sequence that binds to a portion of any one of SEQ ID NOs: 1-7, X2 is a stem sequence the hybridizes to a tracrRNA, Z represents a tracrRNA segment comprising a nucleotide sequence that is partially or completely complementary to X2, and Y represents a linker sequence. In some embodiments, the linker sequence comprises two or more nucleotides and links the crRNA and tracrRNA segments. In some embodiments, the linker sequence comprises 2, 3, 4, 5, 6, 7, 8, 9, 10 or more nucleotides. In some embodiments, the linker is the loop of the hairpin structure formed when the stem sequence hybridized with the tracrRNA. [00199] In some embodiments, a synthetic guide RNA is provided as two separate RNAs where one RNA represents a crRNA segment: 5ʹ-X1-X2-3ʹ where X1 is the targeting sequence that binds to a portion of any one of SEQ ID NOs: 1-7, X2 is a stem sequence the hybridizes to a tracrRNA, and one RNA represents a tracrRNA segment, Z, that is a separate RNA from the crRNA segment and comprises a nucleotide sequence that is partially or completely complementary to X2 of the crRNA. [00200] Exemplary crRNA stem sequences and tracrRNA sequences are provided, for example, in WO/2015/089465, which is incorporated by reference herein. In general, a stem sequence includes any sequence that has sufficient complementarity with a complementary sequence in the tracrRNA to promote formation of a CRISPR complex at a target sequence, wherein the CRISPR complex comprises the stem sequence hybridized to the tracrRNA. In general, degree of complementarity is with reference to the optimal alignment of the stem and complementary sequence in the tracrRNA, along the length of the shorter of the two sequences. Optimal alignment may be determined by any suitable alignment algorithm, and may further account for secondary structures, such as self-complementarity within either the stem sequence or the complementary sequence in the tracrRNA. In some embodiments, the degree of complementarity between the stem sequence and the complementary sequence in the tracrRNA along the length of the shorter of the two when optimally aligned is about or more than about 25%, 30%, 40%, 50%, 60%, 70%, 80%, 90%, 95%, 97.5%, 99%, or higher. In some embodiments, the stem sequence is about or more than about 5, 6, 7, 8, 9, 10, 11, 12, 13, 14, 15, -66- 4892-3380-3674.1
Atty. Dkt. No.: 115872-2919 16, 17, 18, 19, 20, 25, 30, 40, 50, or more nucleotides in length. In some embodiments, the stem sequence and complementary sequence in the tracrRNA are contained within a single RNA, such that hybridization between the two produces a transcript having a secondary structure, such as a hairpin. In some embodiments, the tracrRNA has additional complementary sequences that form hairpins. In some embodiments, the tracrRNA has at least two or more hairpins. In some embodiments, the tracrRNA has two, three, four or five hairpins. In some embodiments, the tracrRNA has at most five hairpins. [00201] In a hairpin structure, the portion of the sequence 5ʹ of the final “N” and upstream of the loop corresponds to the crRNA stem sequence, and the portion of the sequence 3ʹ of the loop corresponds to the tracrRNA sequence. Further non-limiting examples of single polynucleotides comprising a guide sequence, a stem sequence, and a tracr sequence are as follows (listed 5ʹ to 3ʹ), where “N” represents a base of a guide sequence (e.g., a modified oligonucleotide provided herein), the first block of lower case letters represent stem sequence, and the second block of lower case letters represent the tracrRNA sequence, and the final poly-T sequence represents the transcription terminator: (a) NNNNNNNNNNNNNNNNNNNNgtttttgtactctcaagatttaGAAAtaaatcttgcagaagctacaaagataa ggcttcatgccgaaatcaacaccctgtcattttatggcagggtgttttcgttatttaaTTTTTT (SEQ ID NO: 89); (b) NNNNNNNNNNNNNNNNNNNNgtttttgtactctcaGAAAtgcagaagctacaaagataaggcttcatgccg aaatcaacaccctgtcattttatggcagggtgttttcgttatttaaTTTTTT (SEQ ID NO: 90); (c) NNNNNNNNNNNNNNNNNNNNgtttttgtactctcaGAAAtgcagaagctacaaagataaggcttcatgccg aaatcaacaccctgtcattttatggcagggtgtTTTTTT (SEQ ID NO: 91); (d) NNNNNNNNNNNNNNNNNNNNgttttagagctaGAAAtagcaagttaaaataaggctagtccgttatcaactt gaaaaagtggcaccgagtcggtgcTTTTTT (SEQ ID NO: 92); (e) NNNNNNNNNNNNNNNNNNNNgttttagagctaGAAATAGcaagttaaaataaggctagtccgttatcaac ttgaaaaagtgTTTTTTT (SEQ ID NO: 93); and (f) NNNNNNNNNNNNNNNNNNNNgttttagagctagAAATAGcaagttaaaataaggctagtccgttatcaTT TTTTTT (SEQ ID NO: 94).

-67- 4892-3380-3674.1
Atty. Dkt. No.: 115872-2919 [00202] Selection of suitable oligonucleotides for use in as a targeting sequence in a CRISPR Cas system depends on several factors including the particular CRISPR enzyme to be used and the presence of corresponding proto-spacer adjacent motifs (PAMs) downstream of the target sequence in the target nucleic acid. The PAM sequences direct the cleavage of the target nucleic acid by the CRISPR enzyme. In some embodiments, a suitable PAM is 5'- NRG or 5'-NNGRR (where N is any Nucleotide) for SpCas9 or SaCas9 enzymes (or derived enzymes), respectively. Generally the PAM sequences should be present between about 1 to about 10 nucleotides of the target sequence to generate efficient cleavage of the target nucleic acid. Thus, when the guide RNA forms a complex with the CRISPR enzyme, the complex locates the target and PAM sequence, unwinds the DNA duplex, and the guide RNA anneals to the complementary sequence on the opposite strand. This enables the Cas9 nuclease to create a double-strand break. In some embodiments, the sgRNA comprises the nucleic acid sequence of SEQ ID NO: 8, SEQ ID NO: 9, SEQ ID NO: 10, SEQ ID NO: 11, SEQ ID NO: 95, or SEQ ID NO: 96. [00203] A variety of CRISPR enzymes are available for use in conjunction with the disclosed guide RNAs of the present disclosure. In some embodiments, the CRISPR enzyme is a Type II CRISPR enzyme. In some embodiments, the CRISPR enzyme catalyzes DNA cleavage. In some embodiments, the CRISPR enzyme catalyzes RNA cleavage. In some embodiments, the CRISPR enzyme is any Cas9 protein, for instance any naturally-occurring bacterial Cas9 as well as any chimeras, mutants, homologs or orthologs. Non-limiting examples of Cas proteins include Cas1, Cas1B, Cas2, Cas3, Cas4, Cas5, Cas6, Cas7, Cas8, Cas9 (also known as Csn1 and Csx12), Cas10, Csy1, Csy2, Csy3, Cse1, Cse2, Csc1, Csc2, Csa5, Csn2, Csm2, Csm3, Csm4, Csm5, Csm6, Cmr1, Cmr3, Cmr4, Cmr5, Cmr6, Csb1, Csb2, Csb3, Csx17, Csx14, Csx10, Csx16, CsaX, Csx3, Csx1, Csx15, Csf1, Csf2, Csf3, Csf4, homologues thereof, or modified variants thereof. In some embodiments, the CRISPR enzyme cleaves both strands of the target nucleic acid at the Protospacer Adjacent Motif (PAM) site. In some embodiments, the CRISPR enzyme is a nickase, which cleaves only one strand of the target nucleic acid. -68- 4892-3380-3674.1
Atty. Dkt. No.: 115872-2919 [00204] Examples of specific ALAS1-specific inhibitory nucleic acids include givosiran and other RNAi agents disclosed in US Patent No.9,133,461, the contents of which are incorporated herein in their entirety. Modes of Administration and Effective Dosages of the ALAS1/ALAS2-specific Inhibitory Nucleic Acids of the Present Technology [00205] Any method known to those in the art for contacting a cell, organ or tissue with one or more ALAS1/ALAS2-specific inhibitory nucleic acids disclosed herein may be employed. Suitable methods include in vitro, ex vivo, or in vivo methods. In vivo methods typically include the administration of one or more ALAS1/ALAS2-specific inhibitory nucleic acids to a mammal, suitably a human. When used in vivo for enhancing RNAi therapy, the one or more ALAS1/ALAS2-specific inhibitory nucleic acids described herein are administered to the subject in effective amounts (i.e., amounts that have desired therapeutic effect). The dose and dosage regimen will depend upon the degree of the disease state of the subject, the characteristics of the particular ALAS1 or ALAS2-specific inhibitory nucleic acid used, e.g., its therapeutic index, and the subject’s history. [00206] The effective amount may be determined during pre-clinical trials and clinical trials by methods familiar to physicians and clinicians. An effective amount of one or more ALAS1/ALAS2-specific inhibitory nucleic acids useful in the methods may be administered to a mammal in need thereof by any of a number of well-known methods for administering pharmaceutical compounds. The ALAS1/ALAS2-specific inhibitory nucleic acids may be administered systemically or locally. [00207] The one or more ALAS1/ALAS2-specific inhibitory nucleic acids described herein can be incorporated into pharmaceutical compositions for administration, singly or in combination, to a subject as an adjuvant to enhance the efficacy of RNAi therapy. Such compositions typically include the active agent and a pharmaceutically acceptable carrier. As used herein the term “pharmaceutically acceptable carrier” includes saline, solvents, dispersion media, coatings, antibacterial and antifungal agents, isotonic and absorption delaying agents, and the like, compatible with pharmaceutical administration. Supplementary active compounds can also be incorporated into the compositions. -69- 4892-3380-3674.1
Atty. Dkt. No.: 115872-2919 [00208] Pharmaceutical compositions are typically formulated to be compatible with its intended route of administration. Examples of routes of administration include parenteral (e.g., intravenous, intradermal, intraperitoneal or subcutaneous), oral, inhalation, transdermal (topical), intraocular, iontophoretic, and transmucosal administration. Solutions or suspensions used for parenteral, intradermal, or subcutaneous application can include the following components: a sterile diluent such as water for injection, saline solution, fixed oils, polyethylene glycols, glycerine, propylene glycol or other synthetic solvents; antibacterial agents such as benzyl alcohol or methyl parabens; antioxidants such as ascorbic acid or sodium bisulfite; chelating agents such as ethylenediaminetetraacetic acid; buffers such as acetates, citrates or phosphates and agents for the adjustment of tonicity such as sodium chloride or dextrose. pH can be adjusted with acids or bases, such as hydrochloric acid or sodium hydroxide. The parenteral preparation can be enclosed in ampoules, disposable syringes or multiple dose vials made of glass or plastic. For convenience of the patient or treating physician, the dosing formulation can be provided in a kit containing all necessary equipment (e.g., vials of drug, vials of diluent, syringes and needles) for a treatment course (e.g., 7 days of treatment). [00209] Pharmaceutical compositions suitable for injectable use can include sterile aqueous solutions (where water soluble) or dispersions and sterile powders for the extemporaneous preparation of sterile injectable solutions or dispersion. For intravenous administration, suitable carriers include physiological saline, bacteriostatic water, CREMOPHOR EL™ (BASF, Parsippany, N.J.) or phosphate buffered saline (PBS). In all cases, a composition for parenteral administration must be sterile and should be fluid to the extent that easy syringability exists. It should be stable under the conditions of manufacture and storage and must be preserved against the contaminating action of microorganisms such as bacteria and fungi. [00210] The pharmaceutical compositions having one or more ALAS1/ALAS2-specific inhibitory nucleic acids disclosed herein can include a carrier, which can be a solvent or dispersion medium containing, for example, water, ethanol, polyol (e.g., glycerol, propylene glycol, and liquid polyethylene glycol, and the like), and suitable mixtures thereof. The proper fluidity can be maintained, for example, by the use of a coating such as lecithin, by the -70- 4892-3380-3674.1
Atty. Dkt. No.: 115872-2919 maintenance of the required particle size in the case of dispersion and by the use of surfactants. Prevention of the action of microorganisms can be achieved by various antibacterial and antifungal agents, for example, parabens, chlorobutanol, phenol, ascorbic acid, thiomerasol, and the like. Glutathione and other antioxidants can be included to prevent oxidation. In many cases, it will be advantageous to include isotonic agents, for example, sugars, polyalcohols such as mannitol, sorbitol, or sodium chloride in the composition. Prolonged absorption of the injectable compositions can be brought about by including in the composition an agent that delays absorption, for example, aluminum monostearate or gelatin. [00211] Sterile injectable solutions can be prepared by incorporating the active compound in the required amount in an appropriate solvent with one or a combination of ingredients enumerated above, as required, followed by filtered sterilization. Generally, dispersions are prepared by incorporating the active compound into a sterile vehicle, which contains a basic dispersion medium and the required other ingredients from those enumerated above. In the case of sterile powders for the preparation of sterile injectable solutions, typical methods of preparation include vacuum drying and freeze drying, which can yield a powder of the active ingredient plus any additional desired ingredient from a previously sterile-filtered solution thereof. [00212] Oral compositions generally include an inert diluent or an edible carrier. For the purpose of oral therapeutic administration, the active compound can be incorporated with excipients and used in the form of tablets, troches, or capsules, e.g., gelatin capsules. Oral compositions can also be prepared using a fluid carrier for use as a mouthwash. Pharmaceutically compatible binding agents, and/or adjuvant materials can be included as part of the composition. The tablets, pills, capsules, troches and the like can contain any of the following ingredients, or compounds of a similar nature: a binder such as microcrystalline cellulose, gum tragacanth or gelatin; an excipient such as starch or lactose, a disintegrating agent such as alginic acid, Primogel, or corn starch; a lubricant such as magnesium stearate or Sterotes; a glidant such as colloidal silicon dioxide; a sweetening agent such as sucrose or saccharin; or a flavoring agent such as peppermint, methyl salicylate, or orange flavoring. -71- 4892-3380-3674.1
Atty. Dkt. No.: 115872-2919 [00213] For administration by inhalation, the compounds can be delivered in the form of an aerosol spray from a pressurized container or dispenser, which contains a suitable propellant, e.g., a gas such as carbon dioxide, or a nebulizer. Such methods include those described in U.S. Pat. No.6,468,798. [00214] Systemic administration of a therapeutic compound as described herein can also be by transmucosal or transdermal means. For transmucosal or transdermal administration, penetrants appropriate to the barrier to be permeated are used in the formulation. Such penetrants are generally known in the art, and include, for example, for transmucosal administration, detergents, bile salts, and fusidic acid derivatives. Transmucosal administration can be accomplished through the use of nasal sprays. For transdermal administration, the active compounds are formulated into ointments, salves, gels, or creams as generally known in the art. In one embodiment, transdermal administration may be performed by iontophoresis. [00215] A therapeutic agent can be formulated in a carrier system. The carrier can be a colloidal system. The colloidal system can be a liposome, a phospholipid bilayer vehicle. In one embodiment, the therapeutic agent is encapsulated in a liposome while maintaining the agent’s structural integrity. One skilled in the art would appreciate that there are a variety of methods to prepare liposomes. (See Lichtenberg, et al., Methods Biochem. Anal., 33:337-462 (1988); Anselem, et al., Liposome Technology, CRC Press (1993)). Liposomal formulations can delay clearance and increase cellular uptake (See Reddy, Ann. Pharmacother., 34(7-8):915-923 (2000)). An active agent can also be loaded into a particle prepared from pharmaceutically acceptable ingredients including, but not limited to, soluble, insoluble, permeable, impermeable, biodegradable or gastroretentive polymers or liposomes. Such particles include, but are not limited to, nanoparticles, biodegradable nanoparticles, microparticles, biodegradable microparticles, nanospheres, biodegradable nanospheres, microspheres, biodegradable microspheres, capsules, emulsions, liposomes, micelles and viral vector systems. [00216] The carrier can also be a polymer, e.g., a biodegradable, biocompatible polymer matrix. In one embodiment, the therapeutic agent can be embedded in the polymer matrix, while maintaining the agent’s structural integrity. The polymer may be natural, such as polypeptides, -72- 4892-3380-3674.1
Atty. Dkt. No.: 115872-2919 proteins or polysaccharides, or synthetic, such as poly α-hydroxy acids. Examples include carriers made of, e.g., collagen, fibronectin, elastin, cellulose acetate, cellulose nitrate, polysaccharide, fibrin, gelatin, and combinations thereof. In one embodiment, the polymer is poly-lactic acid (PLA) or copoly lactic/glycolic acid (PGLA). The polymeric matrices can be prepared and isolated in a variety of forms and sizes, including microspheres and nanospheres. Polymer formulations can lead to prolonged duration of therapeutic effect. (See Reddy, Ann. Pharmacother., 34(7-8):915-923 (2000)). A polymer formulation for human growth hormone (hGH) has been used in clinical trials. (See Kozarich and Rich, Chemical Biology, 2:548-552 (1998)). [00217] Examples of polymer microsphere sustained release formulations are described in PCT publication WO 99/15154 (Tracy, et al.), U.S. Pat. Nos.5,674,534 and 5,716,644 (both to Zale, et al.), PCT publication WO 96/40073 (Zale, et al.), and PCT publication WO 00/38651 (Shah, et al.). U.S. Pat. Nos.5,674,534 and 5,716,644 and PCT publication WO 96/40073 describe a polymeric matrix containing particles of erythropoietin that are stabilized against aggregation with a salt. [00218] In some embodiments, the therapeutic compounds are prepared with carriers that will protect the therapeutic compounds against rapid elimination from the body, such as a controlled release formulation, including implants and microencapsulated delivery systems. Biodegradable, biocompatible polymers can be used, such as ethylene vinyl acetate, polyanhydrides, polyglycolic acid, collagen, polyorthoesters, and polylactic acid. Such formulations can be prepared using known techniques. The materials can also be obtained commercially, e.g., from Alza Corporation and Nova Pharmaceuticals, Inc. Liposomal suspensions (including liposomes targeted to specific cells with monoclonal antibodies to cell-specific antigens) can also be used as pharmaceutically acceptable carriers. These can be prepared according to methods known to those skilled in the art, for example, as described in U.S. Pat. No.4,522,811. [00219] The therapeutic compounds can also be formulated to enhance intracellular delivery. For example, liposomal delivery systems are known in the art, see, e.g., Chonn and Cullis, “Recent Advances in Liposome Drug Delivery Systems,” Current Opinion in Biotechnology -73- 4892-3380-3674.1
Atty. Dkt. No.: 115872-2919 6:698-708 (1995); Weiner, “Liposomes for Protein Delivery: Selecting Manufacture and Development Processes,” Immunomethods, 4(3):201-9 (1994); and Gregoriadis, “Engineering Liposomes for Drug Delivery: Progress and Problems,” Trends Biotechnol., 13(12):527-37 (1995). Mizguchi, et al., Cancer Lett., 100:63-69 (1996), describes the use of fusogenic liposomes to deliver a protein to cells both in vivo and in vitro. [00220] Dosage, toxicity and therapeutic efficacy of any therapeutic agent can be determined by standard pharmaceutical procedures in cell cultures or experimental animals, e.g., for determining the LD50 (the dose lethal to 50% of the population) and the ED50 (the dose therapeutically effective in 50% of the population). The dose ratio between toxic and therapeutic effects is the therapeutic index and it can be expressed as the ratio LD50/ED50. Compounds that exhibit high therapeutic indices are advantageous. While compounds that exhibit toxic side effects may be used, care should be taken to design a delivery system that targets such compounds to the site of affected tissue in order to minimize potential damage to uninfected cells and, thereby, reduce side effects. [00221] The data obtained from the cell culture assays and animal studies can be used in formulating a range of dosage for use in humans. The dosage of such compounds may be within a range of circulating concentrations that include the ED50 with little or no toxicity. The dosage may vary within this range depending upon the dosage form employed and the route of administration utilized. For any compound used in the methods, the therapeutically effective dose can be estimated initially from cell culture assays. A dose can be formulated in animal models to achieve a circulating plasma concentration range that includes the IC50 (i.e., the concentration of the test compound which achieves a half-maximal inhibition of symptoms) as determined in cell culture. Such information can be used to determine useful doses in humans accurately. Levels in plasma may be measured, for example, by high performance liquid chromatography. [00222] Typically, an effective amount of the one or more ALAS1/ALAS2-specific inhibitory nucleic acids disclosed herein sufficient for achieving a therapeutic or prophylactic effect, range from about 0.000001 mg per kilogram body weight per day to about 10,000 mg per kilogram -74- 4892-3380-3674.1
Atty. Dkt. No.: 115872-2919 body weight per day. Suitably, the dosage ranges are from about 0.0001 mg per kilogram body weight per day to about 100 mg per kilogram body weight per day. For example dosages can be 1 mg/kg body weight or 10 mg/kg body weight every day, every two days or every three days or within the range of 1-10 mg/kg every week, every two weeks or every three weeks. In one embodiment, a single dosage of the therapeutic compound ranges from 0.001-10,000 micrograms per kg body weight. In one embodiment, one or more ALAS1/ALAS2-specific inhibitory nucleic acid concentrations in a carrier range from 0.2 to 2000 micrograms per delivered milliliter. An exemplary treatment regime entails administration once per day or once a week. In therapeutic applications, a relatively high dosage at relatively short intervals is sometimes required until progression of the disease is reduced or terminated, or until the subject shows partial or complete amelioration of symptoms of disease. Thereafter, the patient can be administered a prophylactic regime. [00223] In some embodiments, a therapeutically effective amount of one or more ALAS1/ALAS2-specific inhibitory nucleic acids may be defined as a concentration of inhibitor at the target tissue of 10
-32 to 10
-6 molar, e.g., approximately 10
-7 molar. This concentration may be delivered by systemic doses of 0.001 to 100 mg/kg or equivalent dose by body surface area. The schedule of doses would be optimized to maintain the therapeutic concentration at the target tissue, such as by single daily or weekly administration, but also including continuous administration (e.g., parenteral infusion or transdermal application). [00224] The skilled artisan will appreciate that certain factors may influence the dosage and timing required to effectively treat a subject, including but not limited to, the severity of the disease or disorder, previous treatments, the general health and/or age of the subject, and other diseases present. Moreover, treatment of a subject with a therapeutically effective amount of the therapeutic compositions described herein can include a single treatment or a series of treatments. [00225] The mammal treated in accordance with the present methods can be any mammal, including, for example, farm animals, such as sheep, pigs, cows, and horses; pet animals, such as -75- 4892-3380-3674.1
Atty. Dkt. No.: 115872-2919 dogs and cats; laboratory animals, such as rats, mice and rabbits. In some embodiments, the mammal is a human. [00226] For RNAi therapy applications, a composition comprising an ALAS1/ALAS2-specific inhibitory nucleic acid disclosed herein, is administered to the subject. In some embodiments, the ALAS1/ALAS2-specific inhibitory nucleic acid is administered one, two, three, four, or five times per day. In some embodiments, the ALAS1/ALAS2-specific inhibitory nucleic acid is administered more than five times per day. Additionally or alternatively, in some embodiments, the ALAS1/ALAS2-specific inhibitory nucleic acid is administered every day, every other day, every third day, every fourth day, every fifth day, or every sixth day. In some embodiments, the ALAS1/ALAS2-specific inhibitory nucleic acid is administered weekly, bi-weekly, tri-weekly, or monthly. In some embodiments, the ALAS1/ALAS2-specific inhibitory nucleic acid is administered for a period of one, two, three, four, or five weeks. In some embodiments, the ALAS1/ALAS2-specific inhibitory nucleic acid is administered for six weeks or more. In some embodiments, the ALAS1/ALAS2-specific inhibitory nucleic acid is administered for twelve weeks or more. In some embodiments, the ALAS1/ALAS2-specific inhibitory nucleic acid is administered for a period of less than one year. In some embodiments, the ALAS1/ALAS2- specific inhibitory nucleic acid is administered for a period of more than one year. In some embodiments, the ALAS1/ALAS2-specific inhibitory nucleic acid is administered throughout the subject’s life. [00227] In some embodiments of the methods of the present technology, the ALAS1/ALAS2- specific inhibitory nucleic acid is administered daily for 1 week or more. In some embodiments of the methods of the present technology, the ALAS1/ALAS2-specific inhibitory nucleic acid is administered daily for 2 weeks or more. In some embodiments of the methods of the present technology, the ALAS1/ALAS2-specific inhibitory nucleic acid is administered daily for 3 weeks or more. In some embodiments of the methods of the present technology, the ALAS1/ALAS2- specific inhibitory nucleic acid is administered daily for 4 weeks or more. In some embodiments of the methods of the present technology, the ALAS1/ALAS2-specific inhibitory nucleic acid is administered daily for 6 weeks or more. In some embodiments of the methods of the present -76- 4892-3380-3674.1
Atty. Dkt. No.: 115872-2919 technology, the ALAS1/ALAS2-specific inhibitory nucleic acid is administered daily for 12 weeks or more. In some embodiments, the ALAS1/ALAS2-specific inhibitory nucleic acid is administered daily throughout the subject’s life. Methods for Enhancing the Efficacy of RNAi therapy [00228] In one aspect, the present disclosure provides a method for enhancing efficacy of RNAi therapy in a subject in need thereof, comprising administering to the subject an effective amount of at least one ALAS1-specific inhibitory nucleic acid as disclosed herein and/or at least one ALAS2-specific inhibitory nucleic acid as disclosed herein, wherein the at least one ALAS1- specific inhibitory nucleic acid and/or at least one ALAS2-specific inhibitory nucleic acid inhibits expression levels or activity of 5’-aminolevulinic acid synthase (ALAS) polypeptide in the subject; and administering to the subject an effective amount of at least one RNAi agent that inhibits expression of a disease-inducing gene, wherein the disease-inducing gene is not an ALAS gene. Additionally or alternatively, in some embodiments, the at least one ALAS1- specific inhibitory nucleic acid and/or at least one ALAS2-specific inhibitory nucleic acid is a siRNA, a shRNA, an antisense oligonucleotide, or a sgRNA. In certain embodiments, the at least one ALAS1-specific inhibitory nucleic acid comprises a nucleic acid sequence selected from the group consisting of: SEQ ID NO: 8, SEQ ID NO: 9, SEQ ID NO: 10, SEQ ID NO: 11, SEQ ID NO: 95, SEQ ID NO: 96, or a complement thereof or comprises givosiran. [00229] Additionally or alternatively, in some embodiments of the methods disclosed herein, the at least one RNAi agent comprises a siRNA or a miRNA. In certain embodiments, the miRNA is a tumor-suppressor miRNA. Examples of tumor-suppressor miRNAs include, but are not limited to let-7, miR-15/16, miR-19, miR-34, miR-34a, miR-140, miR-148a, miR-340, miR- 133a-5p, miR-34b-3p, miR-296, miR-188-5p, miR-1291, miR-591, miR-30, miR-188-5p, miR- 34b/c, miR-203, miR141, miR-320, miR-429, and miR-200. In some embodiments, the tumor- suppressor miRNA is a member of a miR family selected from among miR-29 family, let-7 family, mir-30 family and mir-34/449 family. [00230] In any of preceding embodiments of the methods disclosed herein, the at least one ALAS1-specific inhibitory nucleic acid and/or at least one ALAS2-specific inhibitory nucleic -77- 4892-3380-3674.1
Atty. Dkt. No.: 115872-2919 acid is administered orally, topically, intranasally, systemically, intravenously, subcutaneously, intraperitoneally, intradermally, intraocularly, iontophoretically, transmucosally, or intramuscularly. Additionally or alternatively, in some embodiments, the at least one RNAi agent is administered orally, topically, intranasally, systemically, intravenously, subcutaneously, intraperitoneally, intradermally, intraocularly, iontophoretically, transmucosally, or intramuscularly. [00231] Additionally or alternatively, in some embodiments of the methods disclosed herein, administration of the at least one RNAi agent comprises administering an effective amount of an expression vector comprising a nucleic acid sequence encoding the at least one RNAi agent. In certain embodiments, the nucleic acid sequence encoding the at least one RNAi agent is operably linked to a constitutive promoter, an inducible promoter, a tissue-specific promoter or an ubiquitous promoter. [00232] Additionally or alternatively, in certain embodiments of the methods disclosed herein, administration of the at least one ALAS1-specific inhibitory nucleic acid and/or at least one ALAS2-specific inhibitory nucleic acid comprises administering an effective amount of an expression vector comprising a nucleic acid sequence encoding the at least one ALAS1-specific inhibitory nucleic acid or the at least one ALAS2-specific inhibitory nucleic acid. In some embodiments, the nucleic acid sequence encoding the at least one ALAS1-specific inhibitory nucleic acid or the at least one ALAS2-specific inhibitory nucleic acid is operably linked to a constitutive promoter, an inducible promoter, a tissue-specific promoter or an ubiquitous promoter. [00233] In any and all embodiments of the methods disclosed herein, the at least one RNAi agent, the at least one ALAS1-specific inhibitory nucleic acid and/or the at least one ALAS2- specific inhibitory nucleic acid is conjugated to a ligand selected from among a protein, a carbohydrate, a lipid and a drug. Examples of suitable ligands include, but are not limited to human serum albumin (HSA), low-density lipoprotein (LDL), globulin, a dextran, pullulan, chitin, chitosan, inulin, cyclodextrin, hyaluronic acid, lectin, thyrotropin, melanotropin, lectin, glycoprotein, surfactant protein A, mucin carbohydrate, multivalent lactose, multivalent -78- 4892-3380-3674.1
Atty. Dkt. No.: 115872-2919 galactose, N-acetyl-galactosamine, N-acetyl-glucosamine multivalent mannose, multivalent fucose, glycosylated polyaminoacids, multivalent galactose, transferrin, bisphosphonate, polyglutamate, polyaspartate, cholesterol, a steroid, bile acid, folate, vitamin B12, biotin, a RGD peptide or a RGD peptide mimetic. [00234] In any of the above embodiments of the methods disclosed herein, the at least one RNAi agent, the at least one ALAS1-specific inhibitory nucleic acid and/or the at least one ALAS2-specific inhibitory nucleic acid is formulated as a lipid nanoparticle (LNP) or a stable nucleic acid lipid particle (SNALP). [00235] Additionally or alternatively, in some embodiments of the methods disclosed herein, the at least one RNAi agent comprises one or more of patisiran, lumasiran, vutrisiran, inclisiran, cemdisiran, zilebesiran, ALN-XDH, ALN-APP, ALN-HSD, and AB-729. [00236] In any and all embodiments of the methods disclosed herein, the subject suffers from, is diagnosed with, or is at risk for Human papillomavirus (HPV), age-related macular degeneration, Alzheimer’s disease, depression, Huntington’s disease, spinocerebral ataxia, ALS, encephalitis, SARS, influenza, herpes, glioblastoma, prostate cancer, adenocarcinoma, irritable bowel syndrome, RSV, hepatitis B, nonalcoholic steatohepatitis, hypertension, gout, paroxysmal nocturnal hemoglobinuria, polyneuropathy (sensorimotor and autonomic neuropathy), amyloidosis, hereditary transthyretin-mediated amyloidosis, wild-type transthyretin-mediated amyloidosis (ATTRwt), primary hyperoxaluria type 1 (PH1), atherosclerotic cardiovascular disease, rheumatoid arthritis, hypercholesterolemia, pancreatic ductal adenocarcinoma, relapsed or refractory B cell non-Hodgkin lymphoma, dry eye disease, open angle glaucoma, or acute kidney injury. In some embodiments, the subject is human. [00237] Additionally or alternatively, in some embodiments of the methods disclosed herein, the disease-inducing gene is an oncogene, a glycoprotein, an enzyme, a proton-coupled folate transporter, a transport protein, a hormone, or a viral protein. In some embodiments, the disease- inducing gene encodes Transthyretin, glycolate oxidase, PCSK9, Complement component 5, angiotensinogen (AGT), xanthine dehydrogenase (XDH), amyloid precursor protein (APP), or 17β-Hydroxysteroid dehydrogenase type 13. The disease-inducing gene may be expressed in -79- 4892-3380-3674.1
Atty. Dkt. No.: 115872-2919 one or more cell types, including but not limited to, hepatocytes, neurons, astrocytes, microglial cells, retinal pigment epithelial cells, pancreatic ductal cells, blood cells, alveolar cells, tumor cells, intestinal cells, and vaginal epithelial cells. [00238] The at least one ALAS1-specific inhibitory nucleic acid and/or at least one ALAS2- specific inhibitory nucleic acid and the at least one RNAi agent may be administered separately, simultaneously or sequentially. Additionally or alternatively, in some embodiments of the methods disclosed herein, the ALAS1-specific inhibitory nucleic acid and/or ALAS2-specific inhibitory nucleic acid can be administered prior to (e.g., 5 minutes, 15 minutes, 30 minutes, 45 minutes, 1 hour, 2 hours, 4 hours, 6 hours, 12 hours, 24 hours, 48 hours, 72 hours, 96 hours, 1 week, 2 weeks, 3 weeks, 4 weeks, 5 weeks, 6 weeks, 8 weeks, or 12 weeks before), simultaneously with, or subsequent to (e.g., 5 minutes, 15 minutes, 30 minutes, 45 minutes, 1 hour, 2 hours, 4 hours, 6 hours, 12 hours, 24 hours, 48 hours, 72 hours, 96 hours, 1 week, 2 weeks, 3 weeks, 4 weeks, 5 weeks, 6 weeks, 8 weeks, or 12 weeks after) the administration of a RNAi agent to a subject. [00239] In some embodiments, the ALAS1-specific inhibitory nucleic acid and/or ALAS2- specific inhibitory nucleic acid, and RNAi agent are administered to a subject, for example, a mammal, such as a human, in a sequence and within a time interval such that the therapeutic agent that is administered first acts together with the therapeutic agent that is administered second to provide greater benefit than if each therapeutic agent were administered alone. For example, the ALAS1-specific inhibitory nucleic acid and/or ALAS2-specific inhibitory nucleic acid, and RNAi agent can be administered at the same time or sequentially in any order at different points in time; however, if not administered at the same time, the ALAS1-specific inhibitory nucleic acid and/or ALAS2-specific inhibitory nucleic acid, and RNAi agent are administered sufficiently close in time so as to provide the desired therapeutic or prophylactic effect of the combination of the therapeutic agents. In one embodiment, the ALAS1-specific inhibitory nucleic acid and/or ALAS2-specific inhibitory nucleic acid, and RNAi agent exert their effects at times which overlap. In some embodiments, the ALAS1-specific inhibitory nucleic acid and/or ALAS2-specific inhibitory nucleic acid, and RNAi agent each are administered as separate dosage forms, in any appropriate form and by any suitable route. In -80- 4892-3380-3674.1
Atty. Dkt. No.: 115872-2919 other embodiments, the ALAS1-specific inhibitory nucleic acid and/or ALAS2-specific inhibitory nucleic acid, and RNAi agent are administered simultaneously in a single dosage form. [00240] It will be appreciated that the frequency with which any of these therapeutic agents can be administered can be once or more than once over a period of about 2 days, about 3 days, about 4 days, about 5 days, about 6 days, about 7 days, about 8 days, about 9 days, about 10 days, about 11 days, about 12 days, about 13 days, about 14 days, about 20 days, about 28 days, about a week, about 2 weeks, about 3 weeks, about 4 weeks, about a month, about every 2 months, about every 3 months, about every 4 months, about every 5 months, about every 6 months, about every 7 months, about every 8 months, about every 9 months, about every 10 months, about every 11 months, about every year, about every 2 years, about every 3 years, about every 4 years, or about every 5 years. [00241] For example, a ALAS1-specific inhibitory nucleic acid and/or ALAS2-specific inhibitory nucleic acid, or RNAi agent may be administered daily, weekly, biweekly, or monthly for a particular period of time. An ALAS1-specific inhibitory nucleic acid and/or ALAS2- specific inhibitory nucleic acid, or RNAi agent may be dosed daily over a 14 day time period, or twice daily over a seven day time period. An ALAS1-specific inhibitory nucleic acid and/or ALAS2-specific inhibitory nucleic acid, or RNAi agent may be administered daily for 7 days. [00242] Alternatively, an ALAS1-specific inhibitory nucleic acid and/or ALAS2-specific inhibitory nucleic acid, or RNAi agent may be administered daily, weekly, biweekly, or monthly for a particular period of time followed by a particular period of non-treatment. In some embodiments, the ALAS1-specific inhibitory nucleic acid and/or ALAS2-specific inhibitory nucleic acid, or RNAi agent can be administered daily for 14 days followed by seven days of non-treatment, and repeated for two more cycles of daily administration for 14 days followed by seven days of non-treatment. In some embodiments, the ALAS1-specific inhibitory nucleic acid and/or ALAS2-specific inhibitory nucleic acid, or RNAi agent can be administered twice daily for seven days followed by 14 days of non-treatment, which may be repeated for one or two more cycles of twice daily administration for seven days followed by 14 days of non-treatment. -81- 4892-3380-3674.1
Atty. Dkt. No.: 115872-2919 [00243] In some embodiments, the ALAS1-specific inhibitory nucleic acid and/or ALAS2- specific inhibitory nucleic acid, or RNAi agent is administered daily over a period of 14 days. In another embodiment, the ALAS1-specific inhibitory nucleic acid and/or ALAS2-specific inhibitory nucleic acid or RNAi agent is administered daily over a period of 12 days, or 11 days, or 10 days, or nine days, or eight days. In another embodiment, the ALAS1-specific inhibitory nucleic acid and/or ALAS2-specific inhibitory nucleic acid or RNAi agent is administered daily over a period of seven days. In another embodiment, the ALAS1-specific inhibitory nucleic acid and/or ALAS2-specific inhibitory nucleic acid or RNAi agent is administered daily over a period of six days, or five days, or four days, or three days. [00244] In some embodiments, individual doses of the ALAS1-specific inhibitory nucleic acid and/or ALAS2-specific inhibitory nucleic acid, and the RNAi agent are administered within a time interval such that the therapeutic agents can work together (e.g., within 1 hour, 2 hours, 4 hours, 6 hours, 12 hours, 24 hours, 48 hours, 72 hours, 96 hours, 5 days, 6 days, 1 week, or 2 weeks). In some embodiments, the treatment period during which the therapeutic agents are administered is then followed by a non-treatment period of a particular time duration, during which the therapeutic agents are not administered to the subject. This non-treatment period can then be followed by a series of subsequent treatment and non-treatment periods of the same or different frequencies for the same or different lengths of time. In some embodiments, the treatment and non-treatment periods are alternated. It will be understood that the period of treatment in cycling therapy may continue until the subject has achieved a complete response or a partial response, at which point the treatment may be stopped. Alternatively, the period of treatment in cycling therapy may continue until the subject has achieved a complete response or a partial response, at which point the period of treatment may continue for a particular number of cycles. In some embodiments, the length of the period of treatment may be a particular number of cycles, regardless of subject response. In some other embodiments, the length of the period of treatment may continue until the subject relapses. [00245] In some embodiments, the ALAS1-specific inhibitory nucleic acid and/or ALAS2- specific inhibitory nucleic acid is administered for a particular length of time prior to administration of the RNAi agent. For example, in a 21-day cycle, the ALAS1-specific -82- 4892-3380-3674.1
Atty. Dkt. No.: 115872-2919 inhibitory nucleic acid and/or ALAS2-specific inhibitory nucleic acid may be administered on days 1 to 5, days 1 to 7, days 1 to 10, or days 1 to 14, and the RNAi agent may be administered on days 6 to 21, days 8 to 21, days 11 to 21, or days 15 to 21. In other embodiments, the RNAi agent is administered for a particular length of time prior to administration of the ALAS1- specific inhibitory nucleic acid and/or ALAS2-specific inhibitory nucleic acid. For example, in a 21-day cycle, the RNAi agent may be administered on days 1 to 5, days 1 to 7, days 1 to 10, or days 1 to 14, and the ALAS1-specific inhibitory nucleic acid and/or ALAS2-specific inhibitory nucleic acid may be administered on days 6 to 21, days 8 to 21, days 11 to 21, or days 15 to 21. [00246] In one embodiment, the administration is on a 21-day dose schedule in which a once daily dose of ALAS1-specific inhibitory nucleic acid and/or ALAS2-specific inhibitory nucleic acid is administered beginning on day eight for seven days, followed by seven days of non- treatment, in combination with twice-daily administration of the RNAi agent for seven days followed by 14 days of non-treatment (e.g., the ALAS1-specific inhibitory nucleic acid and/or ALAS2-specific inhibitory nucleic acid is administered on days 8-14 and the RNAi agent is administered on days 1-7 of the 21-day schedule). In another embodiment, the administration is on a 21-day dose schedule in which a once daily dose of RNAi agent is administered beginning on day eight for seven days, followed by seven days of non-treatment, in combination with twice-daily administration of the ALAS1-specific inhibitory nucleic acid and/or ALAS2-specific inhibitory nucleic acid for seven days followed by 14 days of non-treatment (e.g., the RNAi agent is administered on days 8-14 and the ALAS1-specific inhibitory nucleic acid and/or ALAS2-specific inhibitory nucleic acid is administered on days 1-7 of the 21-day schedule). Kits [00247] The present disclosure also provides kits comprising one or more ALAS1/ALAS2- specific inhibitory nucleic acids disclosed herein and instructions for using the same to enhance the efficacy of RNAi therapy. In some embodiments, the ALAS1 specific inhibitory nucleic acid is givosiran. Additionally or alternatively, in certain embodiments, the kits further comprise one or more of RNAi agents that inhibit expression of a disease-inducing gene, optionally wherein the disease-inducing gene is not an ALAS gene. -83- 4892-3380-3674.1
Atty. Dkt. No.: 115872-2919 [00248] The above-mentioned components may be stored in unit or multi-dose containers, for example, sealed ampoules, vials, bottles, syringes, and test tubes, as an aqueous, preferably sterile, solution or as a lyophilized, preferably sterile, formulation for reconstitution. The kit may further comprise a second container which holds a diluent suitable for diluting the pharmaceutical composition towards a higher volume. Suitable diluents include, but are not limited to, the pharmaceutically acceptable excipient of the pharmaceutical composition and a saline solution. Furthermore, the kit may comprise instructions for diluting the pharmaceutical composition and/or instructions for administering the pharmaceutical composition, whether diluted or not. The containers may be formed from a variety of materials such as glass or plastic and may have a sterile access port (for example, the container may be an intravenous solution bag or a vial having a stopper which may be pierced by a hypodermic injection needle). The kit may further comprise more containers comprising a pharmaceutically acceptable buffer, such as phosphate-buffered saline, Ringer's solution and dextrose solution. It may further include other materials desirable from a commercial and user standpoint, including other buffers, diluents, filters, needles, syringes, culture medium for one or more of the suitable hosts. The kits may optionally include instructions customarily included in commercial packages of therapeutic or diagnostic products, that contain information about, for example, the indications, usage, dosage, manufacture, administration, contraindications and/or warnings concerning the use of such therapeutic or diagnostic products. [00249] The kit can also comprise, e.g., a buffering agent, a preservative or a stabilizing agent. The kit can also contain a control sample or a series of control samples, which can be assayed and compared to the test sample. Each component of the kit can be enclosed within an individual container and all of the various containers can be within a single package, along with instructions for interpreting the results of the assays performed using the kit. The kits of the present technology may contain a written product on or in the kit container. The written product describes how to use the reagents contained in the kit. In certain embodiments, the use of the reagents can be according to the methods of the present technology. -84- 4892-3380-3674.1
Atty. Dkt. No.: 115872-2919 EXAMPLES [00250] The present technology is further illustrated by the following Examples, which should not be construed as limiting in any way. Example 1. Experimental Materials and Methods [00251] Cell culture [00252] HEK293T cells were grown in a humidified incubator at 37°C, supplemented with 5% CO
2. DME-high glucose media containing 10% fetal bovine serum (FBS), and 100 I.U./mL penicillin/streptomycin was used to culture cells. [00253] CRISPR/Cas9 mutagenesis of heme biosynthesis factors in HEK293T cells [00254] To generate clonal knock-out HEK293T cell lines, cells were transfected with px458 vector encoding GFP, Cas9, and sgRNAs targeting ALAS1 or CPOX. Twenty-four hours after transfection, we collected cells and performed single cell sorting to split the GFP-positive cells into each well of 96-well plates. After two weeks of culturing, the cells were collected for genotyping to identify colonies with the desired indels at the targeted locus. [00255] To generate knockout pools, lentiviral particles were produced by transfecting HEK293T cells with pMD2.G (400 ng/well), psPAX2 (800 ng/well) and lentiCRISPR-v2 encoding Cas9, puromycin-resistance genes, and sgRNAs against target genes using Lipofectamine 2000 (Thermo Fisher) in 6-well plates. After 48hrs transfection, cell culture supernatants were filtered through a 0.45 μm filtration membrane to obtain lentiviral particles. After lentivirus transduction, cells were selected in media containing 1 μg/ml of puromycin for 7 days before further analysis. sgRNA sequences are provided in Table 1. Table 1: Oligonucleotide sequences used in this study Name Sequence (5' -> 3') Note ALAS1- CACCGACAGATCAAAGAAACCCCTC (SEQ ID NO: 8) CRISPR/Cas9 mutagenesis gRNA-1-S ALAS1- AAACGAGGGGTTTCTTTGATCTGTC (SEQ ID NO: 9) CRISPR/Cas9 mutagenesis gRNA-1-AS -85- 4892-3380-3674.1
Atty. Dkt. No.: 115872-2919 ALAS1- CACCGGTGGTTAGTGTGAAAACCGA (SEQ ID NO: 10) CRISPR/Cas9 mutagenesis gRNA-2-S ALAS1- AAACTCGGTTTTCACACTAACCACC (SEQ ID NO: 11) CRISPR/Cas9 mutagenesis gRNA-2-AS UROD-gRNA- CACCGATATCTGGTAGGACAAGTGG (SEQ ID NO: 12) CRISPR/Cas9 mutagenesis S UROD-gRNA- AAACCCACTTGTCCTACCAGATATC (SEQ ID NO: 13) CRISPR/Cas9 mutagenesis AS FECH-gRNA- CACCGTCTGAATAGGAAGTGTCATG (SEQ ID NO: 14) CRISPR/Cas9 mutagenesis S FECH-gRNA- AAACCATGACACTTCCTATTCAGAC (SEQ ID NO: 15) CRISPR/Cas9 mutagenesis AS PPOX-gRNA- CACCGCAGAACAGGTTCCTCTACGT (SEQ ID NO: 16) CRISPR/Cas9 mutagenesis S PPOX-gRNA- AAACACGTAGAGGAACCTGTTCTGC (SEQ ID NO: 17) CRISPR/Cas9 mutagenesis AS CPOX-gRNA- CACCGTAGGAGCATGAGGATTCTTG (SEQ ID NO: 18) CRISPR/Cas9 mutagenesis S CPOX-gRNA- AAACCAAGAATCCTCATGCTCCTAC (SEQ ID NO: 19) CRISPR/Cas9 mutagenesis AS UROS-gRNA- CACCGAAAACTCAAACGATAAAACA (SEQ ID NO: 20) CRISPR/Cas9 mutagenesis S UROS-gRNA- AAACTGTTTTATCGTTTGAGTTTTC (SEQ ID NO: 21) CRISPR/Cas9 mutagenesis AS PBGD-gRNA- CACCGAAAGATGAGAGTGATTCGCG (SEQ ID NO: 22) CRISPR/Cas9 mutagenesis S PBGD-gRNA- AAACCGCGAATCACTCTCATCTTTC (SEQ ID NO: 23) CRISPR/Cas9 mutagenesis AS Control- CACCGGAGGATCCACTAGTCCAGTG (SEQ ID NO: 24) CRISPR/Cas9 mutagenesis gRNA-S Control- AAACCACTGGACTAGTGGATCCTCC (SEQ ID NO: 25) CRISPR/Cas9 mutagenesis gRNA-AS -86- 4892-3380-3674.1
Atty. Dkt. No.: 115872-2919 Ago2-gRNA- CACCGTAACGCCTGCAAGCTCACGC (SEQ ID NO: 26) CRISPR/Cas9 mutagenesis S Ago2-gRNA- AAACGCGTGAGCTTGCAGGCGTTAC (SEQ ID NO: 27) CRISPR/Cas9 mutagenesis AS qhlet7a-F CAACGTAAGTGAATGAAAATGGTC (SEQ ID NO: 28) RT-qPCR qhlet7a-R GAATCACAGTTGAGCACCACAGG (SEQ ID NO: 29) RT-qPCR qh92a-F GGCATAAATACGTGTCTAAATGG (SEQ ID NO: 30) RT-qPCR qh92a-R CACCTCAAACTCTAACAACTATAAACC (SEQ ID NO: 31) RT-qPCR qh16-F TATAGCACTGTGCTGGGCACAG (SEQ ID NO: 32) RT-qPCR qh16-R ACATTATAACATTGATGTAATATGCATAA (SEQ ID NO: 33) RT-qPCR SERTAD3- TGCTGCCAGGTTGCTATAGA (SEQ ID NO: 34) RT-qPCR 3UTR-F SERTAD3- CCGGCCTGGTTATGAAATCA (SEQ ID NO: 35) RT-qPCR 3UTR-R 5SrRNA CCGACCCTGCTTAGCTTCC (SEQ ID NO: 36) Northern Blot probe hsa-miR-16- CGCCAATATTTACGTGCTGCTA (SEQ ID NO: 37) Northern Blot probe 5p hsa-let-7a- AACTATACAACCTACTACCTCA (SEQ ID NO: 38) Northern Blot probe 5p hsa-miR- AAACTCAGTAATGGTAACGGTTT (SEQ ID NO: 39) Northern Blot probe 451-5p hsa-miR- ACAGGCCGGGACAAGTGCAATA (SEQ ID NO: 40) Northern Blot probe 92a-3p hsa-miR- GCGCCCAATTAATGTCTGTTGAT (SEQ ID NO: 41) Northern Blot probe 421-3p mmu-miR- TCAACATCAGTCTGATAAGCTA (SEQ ID NO: 42) Northern Blot probe 21a-5p mmu-miR- ACAACCAGCTAAGACACTGCCA (SEQ ID NO: 43) Northern Blot probe 34a-5p mmu-miR- GGCTGTCAATTCATAGGTCAG (SEQ ID NO: 44) Northern Blot probe 192-5p -87- 4892-3380-3674.1
Atty. Dkt. No.: 115872-2919 mmu-miR- ACAAAGTTCTGTAGTGCACTGA (SEQ ID NO: 45) Northern Blot probe 148a-3p mmu-miR- CAAACACCATTGTCACACTCCA (SEQ ID NO: 46) Northern Blot probe 122-5p 3’ linker /5rApp/NNNNAGATCGGAAGAGCACACGTCT/3ddC/ (SEQ ID NO: 47) Small RNA library preparation 5’ linker ACACUCUUUCCCUACACGACGCUCUUCCGAUCUNNNN (SEQ ID NO: 48) Small RNA library preparation Solexa RT GTGACTGGAGTTCAGACGTGTGCTCTTCCGATCT (SEQ ID NO: 49) Small RNA library preparation Solexa_PCR_ AATGATACGGCGACCACCGAGATCTACACTCTTTCCCTACACGACGCTCTTC Small RNA library CGATCT (SEQ ID NO: 50) preparation F Solexa_PCR_ CAAGCAGAAGACGGCATACGAGATCGTGATGTGACTGGAGTTCAGACGTG Small RNA library TGCTCTTCCGATC (SEQ ID NO: 51) preparation R Index 1 Solexa_PCR_ CAAGCAGAAGACGGCATACGAGATACATCGGTGACTGGAGTTCAGACGTG Small RNA library TGCTCTTCCGATC (SEQ ID NO: 52) preparation R Index 2 Solexa_PCR_ CAAGCAGAAGACGGCATACGAGATTGGTCAGTGACTGGAGTTCAGACGTG Small RNA library TGCTCTTCCGATC (SEQ ID NO: 53) preparation R Index 3 Solexa_PCR_ CAAGCAGAAGACGGCATACGAGATCACTGTGTGACTGGAGTTCAGACGTGT Small RNA library GCTCTTCCGATC (SEQ ID NO: 54) preparation R Index 4 Solexa_PCR_ CAAGCAGAAGACGGCATACGAGATGATCTGGTGACTGGAGTTCAGACGTG Small RNA library TGCTCTTCCGATC (SEQ ID NO: 55) preparation R Index 5 Solexa_PCR_ CAAGCAGAAGACGGCATACGAGATTCAAGTGTGACTGGAGTTCAGACGTGT Small RNA library GCTCTTCCGATC (SEQ ID NO: 56) preparation R Index 6 Pp-luc siRNA pUGUACGCGGAAUACUUCGAUU (SEQ ID NO: 57) In vitro RNAi assay sense Pp-luc siRNA pUCGAAGUAUUCCGCGUACGUG (SEQ ID NO: 58) In vitro RNAi assay antisense L3 linker /5rApp/AGATCGGAAGAGCGGTTCAG/3ddc/ (SEQ ID NO: 59) Ago2 CLIP library preparation cut_oligo_dd GTTCAGGATCCACGACGCTCTTC/3ddC/ (SEQ ID NO: 60) Ago2 CLIP library preparation c (*Pasq) P3 Solexa CAAGCAGAAGACGGCATACGAGATCGGTCTCGGCATTCCTGCTGAACCGCT Ago2 CLIP library CTTCCGATCT (SEQ ID NO: 61) preparation -88- 4892-3380-3674.1
Atty. Dkt. No.: 115872-2919 P5 Solexa AATGATACGGCGACCACCGAGATCTACACTCTTTCCCTACACGACGCTCTTC Ago2 CLIP library CGATCT (SEQ ID NO: 62) preparation RT-1 /5phos/NNaaccNNNAGATCGGAAGAGCGTCGTGgatcCTGAACCGC (SEQ Ago2 CLIP library ID NO: 63) preparation RT-2 /5phos/NNacaaNNNAGATCGGAAGAGCGTCGTGgatcCTGAACCGC (SEQ Ago2 CLIP library ID NO: 64) preparation RT-3 /5phos/NNattgNNNAGATCGGAAGAGCGTCGTGgatcCTGAACCGC (SEQ Ago2 CLIP library ID NO: 65) preparation RT-4 /5phos/NNaggtNNNAGATCGGAAGAGCGTCGTGgatcCTGAACCGC (SEQ Ago2 CLIP library ID NO: 66) preparation SERTAD3- AAAGCGGCCGCGTTACCCCGACCTTCTGTGA (SEQ ID NO: 67) Sensor construct cloning UTR-F(NotI) SERTAD3- AAACTCGAGAAAGCCACCACAAACTCTGC (SEQ ID NO: 68) Sensor construct cloning UTR-R(XhoI) pri-miR-375- AAAGCGGCCGCGACGTGTCAGCCGCAGAT (SEQ ID NO: 69) Sensor construct cloning F(NotI) pri-miR-375- AAACTCGAGCCCGTATTACGACGCAGAAT (SEQ ID NO: 70) Sensor construct cloning R(XhoI) 451BS- AAACTGGGGCACAGATAAATGAGTCGACAAAAAACTCAG (SEQ ID NO: 71) tRNA-miR-451 reporter generation pLenti-F 451BS- CAGAGGTTGATTGTTCCAGAACTAGTCTCGAGTTTTAAAC (SEQ ID NO: 72) tRNA-miR-451 reporter generation pLenti-R tRNAlys_451 GCCCGGATAGCTCAGTCGGTAGAGCATCAGACTTTTAATCTGAGGGTCCAG tRNA-miR-451 reporter GGTTCAAGTCCCTG (SEQ ID NO: 73) generation _S tRNAlys_451 AAAAACCATTACCATTACTAAACTCAGTAATGGTAACGGTTTCGCCCGAACA tRNA-miR-451 reporter GGGACTTGAACCC (SEQ ID NO: 74) generation _AS tRNAlys-451- CCGATGATTAATTGTCAACACGTGCTGCAGGTCCGAGGTTAGGTTCTAGAT tRNA-miR-451 reporter GCATGCTCGAGC (SEQ ID NO: 75) generation pLenti-F tRNAlys-451- ACCCCGTAATTGATTACTATTAATAACTAATGCATGGCGAGTAACGGCCGCC tRNA-miR-451 reporter AGTGTGCT (SEQ ID NO: 76) generation pLenti-R siGFP-S pGGCAAGCUGACCCUGAAGUUC (SEQ ID NO: 77) synthetic siRNA siGFP-AS pACUUCAGGGUCAGCUUGCCGU (SEQ ID NO: 78) synthetic siRNA N1 TGCGAGGCCAGAGGCCACTTGTGTAGC (SEQ ID NO: 79) sequencing primers for ALAS1-LoxP targeting vector -89- 4892-3380-3674.1
Atty. Dkt. No.: 115872-2919 N3 ATCACCTGACAACTCACTCC (SEQ ID NO: 80) sequencing primers for ALAS1-LoxP targeting vector T73 TAATGCAGGTTAACCTGGCTTATCG (SEQ ID NO: 81) sequencing primers for ALAS1-LoxP targeting vector P6 GAGTGCACCATATGGACATATTGTC (SEQ ID NO: 82) sequencing primers for ALAS1-LoxP targeting vector F1530specS GAGTCGTTCTGAAGCCAGTTGG (SEQ ID NO: 83) sequencing primers for ALAS1-LoxP targeting vector SC1 GCAGCCTCCGTAGGCACTGCTGTC (SEQ ID NO: 84) primers to confirm recombinant clones for ALAS1-LoxP targeting vector LAN1 CCA GAG GCC ACT TGT GTA GC (SEQ ID NO: 85) primers to confirm recombinant clones for ALAS1-LoxP targeting vector A1 CCA AAG CTC AGC GTG AAA GG (SEQ ID NO: 86) primers to confirm recombinant clones for ALAS1-LoxP targeting vector LUNI GCA TCG CCT TCT ATC GCC TTC TTG (SEQ ID NO: 87) primers to confirm recombinant clones for ALAS1-LoxP targeting vector SDL2 CAA AAC CAA CAC ATA ACT CAT TGC (SEQ ID NO: 88) primers to confirm recombinant clones for ALAS1-LoxP targeting vector [00256] Luciferase sensor assays [00257] Full-length of SERTAD33’-UTR and pri-miR-375 sequences were PCR amplified from HEK293T genomic DNA and cloned into psiCHECK2 luciferase sensor plasmid. Generation of four-copy-site bulge targets of miR-451-5p sensor was described previously (PMID: 20699384). Primers used for cloning are listed in Table 1. [00258] HEK293T cells were seeded per well of 96-well plate and transfected with total 120ng of plasmids including psiCHECK2 sensor. Luciferase were measured 2 days after transfection using Dual Glo luciferase assay system (Promega) and Cytation5 (BioTek). For -90- 4892-3380-3674.1
Atty. Dkt. No.: 115872-2919 tRNA
lys-miR-451 sensor assay, the fold repression values were normalized to luciferase activity measured by expression of the sensor co-transfected with tRNA
lys-miR-155. Individual tests were done in quadruplicate and the averaged values from three biological replicate samples were subjected to statistical analysis. [00259] qPCR [00260] Total RNA was extracted using Trizol (Invitrogen) according to the manufacturer’s instruction. RNA samples were then converted to cDNA using SuperScript™ III Reverse Transcriptase (Invitrogen) using Random Hexamers (Thermo Fisher Scientific). Quantitative PCR (qPCR) was performed in the BioRad CFX96 Real-time PCR system. SYBR™ Select Master Mix (Applied Biosystems) was used to measure expression of mRNAs with gene-specific primers listed in Table 1. [00261] Antibodies [00262] The primary antibodies used were anti-ALAS1 (Abcam #ab154860), anti- CPOX(Abcam, ab169766), anti-GAPDH(Cell Signaling, #2118), and anti-Ago2(Abnova, #H00027161-M01). [00263] Small RNA Northern blotting [00264] A 12% Urea acrylamide gel (SequaGel UreaGel System, National Diagnostics) in 1X TBE buffer was used to resolve small RNAs, which was then transferred to a GeneScreen Plus membrane (PerkinElmer) and UV-crosslinked. The membrane was subsequently hybridized with 5’ end labeled oligonucleotides labeled with (g-32P) ATP and incubated overnight at 42°C. Following this, the membrane was exposed to a phosphorimaging plate (Fujifilm) and analyzed using the BAS-3000 system (Fujifilm). Sequences of Northern blot probes are listed in Table 1. [00265] Analysis of RNA chemical structure [00266] To analyze 3′ of RNA termini, β-elimination reaction was performed with RNA. 10 μl of 61.5 mM NaIO4 in borax/boric-acid buffer was incubated with 10ug of total RNA for 30min at room temperature. 2.5 μl of 500 mM NaOH was added and the incubation process was prolonged for 90 minutes at 45 °C. To analyze 5′ of RNA termini, RNA samples were incubated -91- 4892-3380-3674.1
Atty. Dkt. No.: 115872-2919 with 2 units CIP (New England Biolabs) in 1× buffer 3 (NEB, 100 mM NaCl, 50 mM Tris-HCl, 10 mM MgCl2, 1 mM dithiothreitol) for 2 h at 37 °C. RNA was purified by phenol/chloroform extraction followed by ethanol precipitation for subjected to Northern blotting analysis. [00267] Small RNA sequencing libraries [00268] To analyze small RNAs, we isolated biologically independent total RNA samples from HEK293T, ALAS1
-/- cells using Trizol (Invitrogen). One milligram of total RNA was used to generate small RNA libraries for each sample. After adding 1 µL of QIAseq TM miRNA Library QC Spike-Ins (QIAGEN) to the total RNA, a 3’ linker was ligated to it in a reaction containing 100 mM 3’ linker, T4 RNA Ligase 2, truncated KQ (NEB), 10% PEG8000, 1X RNA ligation buffer, and 20 U RNase OUT overnight at 17°C. The 48-57 nucleotide sized 3’ linker- ligated small RNAs were purified using 12% Urea-PAGE gel with radio-labeled oligonucleotides serving as size-markers. Subsequently, the small RNA-linker hybrid was subjected to a 5’ ligation reaction overnight at 17°C containing 100 mM 5’ adaptor, 20% PEG8000, 1X RNA ligase buffer, 1 mM ATP, 20 U RNaseOUT, and 5 U T4 RNA ligase 1. The linker-ligated RNAs were then reverse transcribed, PCR amplified using 12 cycles of PCR with forward and Illumina index reverse primers, and purified by 8% non-denaturing acrylamide gel. Sequencing was performed with HiSeq 4000 in SE50 mode. Oligo sequences used for library preparation are provided in Table 1. [00269] After removing adaptor sequences of the small RNA sequencing libraries using Cutadapt, the reads were collapsed subsequently to remove PCR artifacts using fastx_collapser, and 5’ and 3’ end linker sequences were clipped using fastx_clipper. The remaining small RNA reads were mapped to the hg38 genome assembly, and small RNA counts are normalized by spike-in reads in each library. Differential small RNA expression analysis was performed by Bioconductor R package Limma. [00270] RISC assembly assay [00271] HEK293T cells were lysed with 100 mM potassium acetate, 30 mM HEPES-KOH, pH 7.4, 2 mM magnesium acetate containing 5 mM dithiothreitol (DTT) and protease inhibitor cocktails (Roche) at 4°C, followed by centirifugation for 15 min at 14,500g. For each sample, -92- 4892-3380-3674.1
Atty. Dkt. No.: 115872-2919 lysate were incubated with 32p-labeled siRNA duplexes containing a G:U wobble at the 5’ end of the sense strand supplemented with 0.03 μg/ml creatine kinase, 25 mM creatine phosphate, 1 mM ATP, and 10% glycerol. Reactions were quenched by adding 2 μl of a heparin mix (60 mM potassium phosphate, 3 mM magnesium chloride, 3% PEG8000, 8% glycerol, 4 mg/ml heparin). RISC assembly was visualized by native gene electrophoresis using 4% (40:1 acrylamide:bisacrylamide) native gel and run at 4°C in 1× TBE at 10W. [00272] Ago2 CLEAR CLIP [00273] One 25cm dish of confluent HEK293T and ALAS1
-/- cells were used per sample. The cells were UV irradiated with 150mJ/cm
2 UVC (254nM wavelength) two times. The cells were then lysed on ice with iCLIP lysis buffer (50 mM Tris-HCl pH7.4, 100 mM NaCl, 1% NP- 40, 0.1% SDS, 0.5% SDC, protease inhibitor cocktail (Roche), and 40 U/ml RNaseOUT (Sigma)) for 20min on ice. TurboDNase (Invitrogen #AM2238, 10U), RNase I (0.05 U/mL) were added subsequently, and samples were incubated at 37°C for 5 min. Treated lysates were immunoprecipitated with anti-Ago2 antibodies (Abnova) or control IgG (MilliporeSigma) coupled with Dynabeads protein G (Thermo Fisher Scientific) for 2 hrs at 4°C with gentle agitation. The immunoprecipitate was washed sequentially with high-stringency buffer, high-salt buffer (50 mM Tris-HCl pH 7.4, 1 M NaCl, 1 mM EDTA, 1% Nonidet P-40, 0.5% sodium deoxycholate, and 0.1% SDS), and PNK wash buffer (20 mM Tris-HCl pH 7.4, 10 mM MgCl2, and 0.2% Tween-20). Samples were then phosphorylated at 37°C for 20 minutes in 50 µl of PNK mixture: 41.8µl H
2O, 5 µl 10X PNK buffer (NEB), 1 µl RNaseOUT, 1.67 µl ATP (30 mM), 0.5 µl T4 PNK - 3’ phosphatase minus (NEB M0236L). The immunoprecipitated were washed the PNK wash buffer then miRNA-mRNA ligation was carried out overnight at 17°C in 100µL of mixture: 49.25 µl H
2O, 30 µl 50% PEG-8000 (NEB #B1004) 10 µl 10X T4 RNA ligation buffer (NEB), 2.5 µl RNaseOUT, 1 µl ATP (100mM), 1 µl BSA (10 mg/ml), 6.25 µl T4 RNA ligase 1 (10U/µl – NEB M0204). The RNAs in the immunoprecipitate were dephosphorylated on the beads with FastAP Thermosensitive alkaline phosphatase (Thermo Fisher Scientific) and ligated to preadenylated adaptors in 40 µL of mixture: 17µl H
2O, 4µl 10X T4 RNA ligase buffer (NEB), 1µl of 3’linker, 16µl 50% PEG-8000, 1 µl RNaseOUT and 1µl T4 -93- 4892-3380-3674.1
Atty. Dkt. No.: 115872-2919 RNA ligase 2 truncated K227Q (NEB M0351) at room temperature for 5 hrs. Samples were then radiolabeled in 50µl mixture: 5 µl 10X PNK buffer (NEB), 1 µl RNaseOUT, 1.5 µl g-p32-ATP (15μCi), 1 µl PNK enzyme (NEB M0201) and 41.5 µl H
2O for 5 min at 37°C. Protein–RNA complexes were eluted from the beads, electrophoresed on 4–20% Bis-Tris NuPAGE gels (Thermo Fisher Scientific), transferred to nitrocellulose membranes (Whatman), and visualized by autoradiography. The area of the membrane corresponding with proteins that were ;100 kDa (the MW of Ago2) to 150 kDa in size was excised from each lane of the blot. Excised lanes were treated with proteinase K (MilliporeSigma) and 7 M urea (MilliporeSigma). The RNA- adaptor products were purified by acid phenol:chloroform: isoamyl alcohol extraction and ethanol precipitation. Purified RNAs were then undergo reverse transcription using Superscript III (Thermo Fisher) with adapter-specific RT primers according to the manufacturer’s instruction. Resulted single-stranded cDNA products were purified using ExoSAP-IT (Thermo Fisher Scientific), followed by circularization using CircLigase (Illumina), and PCR-amplified. The resulting libraries were gel-purified and sequenced with the Illumina HiSeq platform. Oligonucleotides used for library preparation are provided in Table 1. [00274] Generation of HEK293T tRNA
lys-miR-451 reporter cell lines [00275] RFP and four-bulge-target site of miR-451-5p were PCR amplified and cloned downstream of CMV promoter and tRNA
lys-miR-451 sequences were synthesized by IDT and PCR amplified to be cloned in opposite direction of the CMV promoter into the pLenti-CMV backbone using GIBSON assembly kit (NEB). Lentivirus was generated using the plasmid, and recipient HEK293T cells were transduced at a multiplicity of infection (MOI) of ~0.1. RFP positive cells were sorted by FACS followed by derivation of clonal lines. Each of the single- cell derived clones were validated with their tRNA
lys-miR-451 expression by Northern blotting and of those reporter sensitivity was validated by inducing heterogenous Ago2 CRISPR-Cas9 knockout of single clones. One most sensitive reporter clone was used for downstream experiments. Oligonucleotides used to generated reporter cell lines are provided in Table 1. [00276] siRNA transfection -94- 4892-3380-3674.1
Atty. Dkt. No.: 115872-2919 [00277] Stable GFP expressing HEK293T and ALAS1-/- cells were pre-seeded in 24-well plate one day prior to transfection and GFP siRNA complexes were added into transfection media with final siRNA concentration 10nM using Lipofectamine RNAiMAX Transfection Reagent (Invitrogen). Cells were collected and GFP expression was analyzed after indicated hours of transfection. [00278] Generation of inducible conditional Alas1 knockout mouse line [00279] The Alas1-LoxP mouse line in which Alas1 exons 4 and 5 are flanked by tandemly- oriented LoxP sites was generated by ingenious Targeting Laboratory (iTL). A targeting vector, as depicted in FIG.20A, was constructed using mouse BAC cloneRP23-441D9 (chr9, 105969973~106174967). PCR primers that contain the flanking genomic sequences for the iNeo cassette and Spec cassette PCR syntheses were generated, and the PCR products were used for bacterial homologous recombination (BHR) to insert the cassettes into the BAC clone respectively. The recombinants were screened and confirmed by colony PCR and sequencing using primer sequences provided in Table 1. In order to reduce the targeting vector size and provide more convenience for ES clone screening by PCR and Southern Blot, a backbone vector [3948bp, modified from pSP72 (Promega) by iTL, containing DT (Diphtheria toxin) cassette] to subclone the target fragment flanked by two homology arms, LA - LoxP_Spec cassette - MA - iNeo cassette - SA (LA: long arm; SA short arm; MA: middle arm) was designed. The same method as described above was used for BHR to subclone the targeted fragment from the BAC clone. Finally, the Spec was removed by MluI digestion and self-ligation. To confirm proper assembly of the targeting vector, junction regions were PCR amplified and sequenced after each BHR step. The final targeting vector was confirmed by sequencing and by restriction enzyme digestions. [00280] Ten micrograms of the targeting vector was linearized by NotI and then transfected by electroporation of C57Bl/6 (B6) embryonic stem cells. After selection with G418 antibiotic, surviving clones were expanded for PCR analysis to identify recombinant ES clones using primer sequences provide in Table 1. Positive clones were expanded and confirmed by PCR and Southern blotting (FIGs.20C-20D) and checked for correct karyotype. Select clones were -95- 4892-3380-3674.1
Atty. Dkt. No.: 115872-2919 injected into blastocysts. Heterozygous founder mice were bred against FLP Deleter mice to excise the neomycin cassette from all tissues. The resulting mice were then bred against each other to produce mice that have floxed Alas1 on both alleles (Alas1-LoxP mice). [00281] Generation of TMX-inducible hepatocyte-specific Alas1 knockout mice. [00282] To generate TMX- inducible hepatocyte-specific Alas1KO mice, Alas1-LoxP mice were bred against Alb-Cre-ERT2 mice
1 (Schuler M, Dierich A, Chambon P, Metzger D. Genesis. 2004;39(3):167-172) using mating pairs Alas1-LoxP
+/+/Cre-ERT2
-/- x Alas1-LoxP
+/+/Cre- ERT2
+/-. The resulting Alas1-LoxP
+/+/Cre-ERT2
+/- mice (designated iAlas1KO mice) were bred against the mt/mg reporter mouse line (Muzumdar MD, Tasic B, Miyamichi K, Li L, Luo L. Genesis.2007;45(9):593-605). Experimental mice were Alas1-LoxP
+/+/Cre-ERT2
+/-/mtmg
+/- mice, produced by breeding Alas1-LoxP
+/+/Cre-ERT2
+/-/mtmg
+/- mice against Alas1- LoxP
+/+/Cre-ERT2
-/-/mtmg
-/- mice. [00283] Generation of mouse lines in which Alas1 knockout can be induced in neurons (CNS), astrocytes (CNS), microglia (CNS), retinal pigmental epithelium (eye), pancreatic ductal cells, and alveolar cells (lungs). [00284] Alas1-LoxP mice were bred against mouse strains: 1) B6;129S6- Prkcgtm2
(cre/ERT2)Ddg/J (Jax strain#030289), 2) B6N.FVB-Tg
(Aldh1I1-cre/ERT2)1Khakh/J (#031008), 3) C57BL/6-Tmem119
em1(cre/ERT2)Ghng/J (#031820), 4) C57BL/6-Rpe65
tm1.1(Cre/ERT2)Kser /J (#035973), 5) Tg
(Sox9-cre/ERT2)1Msan/J (#018829), or 6) B6.129S-Sftpc
tm1(cre/ERT2)Blh/J (#028054) to generate mice with TMX-inducible Alas1 knockout in neurons, astrocytes, microglia, retinal pigmental epithelium, pancreatic duct cells, or alveolar cells. The resulting mice were subsequently bred against the mt/mg reporter mice using a similar breeding scheme as described above so that cre-expressing cells are GFP-positive and readily identified. [00285] Mouse studies [00286] Mice were housed in standard cages in a barrier facility at the Icahn School of Medicine at Mount Sinai (ISMMS) Center for Comparative Medicine and Surgery, maintained at 22℃ on a 12-12 hour light-dark cycle. All mouse experiments were performed in accordance -96- 4892-3380-3674.1
Atty. Dkt. No.: 115872-2919 with institutional guidelines and regulations and were approved by the ISMMS Institutional Animal Care and Use Committee. [00287] All studies utilized 1-3 month old male and female mice. On days 1-3, TMX was administered (0.5 mg/kg/day; i.p.) to the iAlas1KO mice to ‘knockout’ Alas1 expression in the specific cell type (e.g., hepatocytes, neurons, astrocytes, microglia, retinal pigmental epithelium, pancreatic ductal cells, or alveolar cells) generating various Alas1KO mouse lines. Hepatocytes unexpectedly expressed high levels of Alas2 mRNA. Therefore, a hepatocyte-specific Alas1/2KO mouse model was generated by administering into hepatocyte-specific Alas1KO mice a single dose of GalNAc-conjugated siRNA targeting hepatocyte Alas2 (10mg/kg, s.c.) on the same day as the last TMX dose (day 3). [00288] Mice were euthanized at the indicated times, after being anesthetized with ketamine/xylazine (100/5 mg/kg, i.p.) and given a toe pinch test to ensure sufficient anesthetization. For whole tissue experiments, mice were perfused with 1x phosphate-buffered saline (PBS) via the left cardiac ventricle, and tissues, including liver, kidney, heart, brain, lung and pancreas were harvested and stored at -80ºC until use. Hepatocyte isolations were performed as described in the following section. [00289] Demonstration of enhanced siRNA-mediated silencing in Alas1/2KO hepatocytes [00290] For experiments in which hepatocyte-specific Alas1/2KO mice were administered a non-Alas-siRNA to demonstrate enhancement of gene silencing, a single dose of GalNAc- conjugated siRNA (e.g., siRNA targeting Slc46A1) was administered 48 h following the last TMX and/or Alas2-siRNA dose. Administration doses of the non-Alas-siRNAs (e.g., Slc46A1- targeted siRNA) were optimized so that they knocked down target gene expression by 25-50% in hepatocytes in the absence of ALAS suppression/depletion. Hepatocytes were isolated 24 h following administration of the non-Alas-siRNA and assessed for mRNA levels of the target gene (e.g., Slc46A1) by qPCR, as described herein. [00291] Generation of T1T2 control livers for western blots -97- 4892-3380-3674.1
Atty. Dkt. No.: 115872-2919 [00292] To obtain the T1T2 mouse liver samples used as controls for ALAS1 Western blots, T1T2 mice were administered phenobarbital (110, 120, 130 mg/kg/day; i.p.) with or without an LNP-conjugated Alas1-siRNA (1.0 mg/kg; i.v.), using previously described procedures. [00293] Hepatocyte Isolation by Differential Centrifugation [00294] Mice were deeply anesthetized with ketamine/xylazine (100/5 mg/kg, i.p.), and a lateral incision was made through the integument and abdominal wall up to the ribcage. The diaphragm and pleural cavities were incised, a 25G i.v. catheter was inserted into the hepatic portal vein, and the inferior vena cava was cut to create an outlet in the circulatory system for exsanguination. Liver Perfusion medium (30 mL), heated to 37ºC, was pumped through the catheter line using a Gilson Minipuls 2 peristaltic pump at a rate of 6.5 mL/min, followed by 30 mL of 37ºC Hepatocyte Wash medium containing Collagenase B (0.05% Collagenase B solution). Liver tissue was excised, transferred to a petri dish containing 5 mL of liver digestion buffer (0.05% Collagenase B solution diluted 10X in Hepatocyte Wash medium), and incubated for 10-13 min at 37ºC. Digested liver tissue was filtered through a 70 µm cell strainer and then centrifuged at 50 x g for 5 min at 4ºC to isolate hepatocytes. The hepatocytes were washed three times using Hepatocyte Wash medium and centrifugation at 50 x g for 5 min at 4ºC. [00295] PCR Assays for Genotyping and for Evaluation of Excision of Alas1 exons 4/5 [00296] Genotypes were determined using tail clips collected from mice within the first week of birth. Genomic DNA (gDNA) was isolated using the Extract-N-Amp Tissue™ PCR Kit, according to the manufacturer’s protocol, from tail clips (for genotyping) or tissues (for Alas1 exons 4/5 excision analyses). DNA concentrations were determined using the NanoDrop ND- 1000 UV/VIS Spectrophotometer. PCR conditions used for: 1) genotyping the Cre-ERT2 allele, 2) genotyping the mt/mg allele, and 3) evaluation of Alas1 exons 4/5 excision are provided in Table 2. -98- 4892-3380-3674.1
Atty. Dkt. No.: 115872-2919 [00297] Table 2
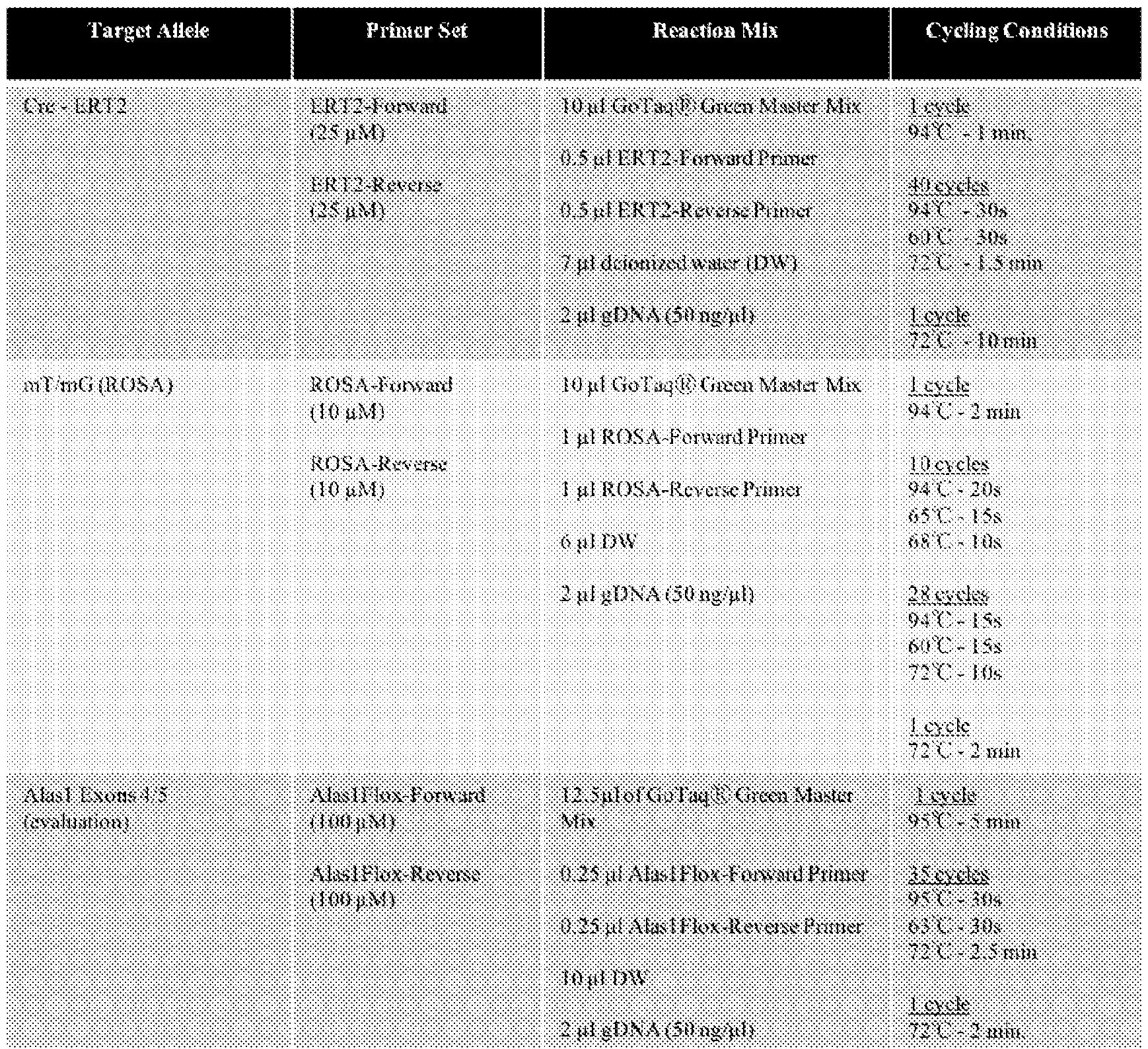
The full PCR reaction volume was run on a 1.5% Tris/Borate/EDTA (TBE) agarose gel with 5 µl ethidium bromide (10 mg/mL) at 150V. Gel images were captured using the Molecular Imager Ⓡ GelDoc™ XR+ and ImageLab™ software. [00299] Relative mRNA quantification using a Taqman-based Quantitative PCR (qPCR) Assay [00300] Alas1 and Alas2 mRNA levels were quantified using previously described methods (Yasuda M, Gan L, Chen B, Kadirvel S, Yu C, Phillips JD, New MI, Liebow A, Fitzgerald K, -99- 4892-3380-3674.1
Atty. Dkt. No.: 115872-2919 Querbes W, and Desnick RJ. Proc Natl Acad Sci USA 111: 7777-7782, 2014). Total RNA was isolated from tissue (~0.3 g) or hepatocytes using TRI Reagent and the manufacturer’s protocol. Total RNA (1 µg) was reverse transcribed using SuperScript™ II Reverse Transcriptase. qPCR was performed using pre-designed Taqman assays [β-actin (Assay ID: Mm02619580), Alas1 (Mm01235914), Alas2 (Mm00802083)]. Each sample was prepared in triplicate in a 96-well plate and was composed of 10 µl Taqman Universal PCR Master Mix, 1 µl of the corresponding FAM-labeled probe/primer mix, 2 µl of sample cDNA (80 ng total) and 7 µl DEPC-treated water for a final 20 µl reaction. Plates were covered with a plastic seal and aluminum foil to block light and kept frozen until use. PCR amplification was performed using standard conditions (50ºC for 2 minutes, 95ºC for 10 minutes, followed by 40 cycles of 95℃ for 15s and 60℃ for 60s). Ct values were generated by the Applied Biosystems ViiA™ 7 Real-Time PCR System with QuantStudio™ RT-PCR Software v1.3. Relative mRNA expression levels were calculated using the delta-delta Ct method
5, by normalizing against expression of β-actin. [00301] Bradford Assay for Protein Quantification [00302] Protein concentrations were measured in liver tissue or hepatocytes using the Bradford method
6. Bovine Serum Albumin (BSA) Standards (1, 0.5, 0.25, 0.125 and 0.0625 mg/mL) were generated by diluting a 10 mg/mL stock solution. 10 µl of each standard or test sample was loaded into a clear, flat-bottom 96-well plate and 200 µl of Bio-Rad Protein Assay Dye Reagent was added to each well. Following a 5 min incubation at room temperature, absorbances were measured (595 nm excitation) using the Synergy™ H1 microplate reader. Test sample concentrations were calculated using the linear regression of the standard curve. [00303] Western Blot Analysis to Quantitate ALAS1 Protein in Murine Tissues [00304] Western blot analyses were performed as previously described
4. Briefly, tissues were homogenized on ice in lysis buffer solution [20 mM Hepes, pH 7.4, 150 nM NaCl, 0.5% octylphenoxypolyethoxyethanol (IGEPAL) with Pierce™ Protease Inhibitor Mini Tablet]. Lysates (~60 µg protein per sample) were diluted to a final volume of 20 µl with NuPAGE™ LDS Sample Buffer, heated at 100ºC for 5 min, then loaded onto NuPAGE™ (4-12%) Bis-Tris polyacrylamide gels (4-12%) for sodium dodecyl sulfate-polyacrylamide gel electrophoresis -100- 4892-3380-3674.1
Atty. Dkt. No.: 115872-2919 (SDS-PAGE; 100 V for 2 hours in NuPAGE™ MOPS SDS Running Buffer). Following electrophoresis, proteins were transferred onto a polyvinylidene difluoride (PVDF) blotting membrane at 100 V for 1.5 hrs at 4ºC in transfer buffer (0.12M tris, 0.04M glycine, and 20% methanol). Membranes were blocked with 5% skim milk in tris-buffered saline with 0.1% TweenⓇ 20 [(TBST), 0.1M Tris-Cl, pH 7.5, 0.15M NaCl, 0.1% Tween 20] for 1 h and then incubated with a rabbit polyclonal anti-ALAS1 antibody (1:1000 dilution in 5% skim milk/TBST) overnight at 4℃ with continuous shaking. Following washing, membranes were incubated with IRDye 800CW goat anti-rabbit secondary antibody (1:25,000 dilution in 5% skim milk/TBST) for 1 h at room temperature in the dark. Signals were detected using the Odyssey Clx by LI-COR. Membranes were stripped using Restore™ PLUS Western Blot Stripping Buffer for 30 min, blocked with 5% skim milk/TBST, and then re-probed with horseradish peroxidase (HRP)-conjugated β-Actin monoclonal antibody (1:3,000 dilution in 5% skim milk/TBST). Washed membranes were exposed to SuperSignal™ West Atto Ultimate Sensitivity Chemiluminescent Substrate for immediate imaging using FluorchemⓇQ MultiImagerIII. [00305] Quantitation of ALAS Enzymatic Activity in Murine Tissues [00306] ALAS enzymatic activities were measured in murine tissues by the Iron and Heme Core Facilities at the University of Utah using previously described methods. PBS-perfused frozen tissue samples (~100 mg) were homogenized with 0.25 M sucrose. Protein concentrations were determined for each sample and adjusted to 12 mg/mL in 0.125 M sucrose on ice. To inhibit ALAD activity, succinyl acetone was added to each sample to a final concentration of 50 µM. 25 µl protein samples were combined 1:1 with assay buffer for a final concentration of 50 mM glycine, 100 µM succinyl-CoA, 80 µM pyridoxal phosphate, 50 µM succinyl acetone and 25 mM potassium phosphate (pH 7.4). This mixture was incubated at 37℃ for 30 min. After stopping the enzymatic reaction by adding 450 µl ice-cold water, ALA was derivatized by adding 150 µl derivatizing agent (water, formaldehyde, ethanol and acetylacetone at a 107:5:15:23 ratio), and vortexing. This mixture was incubated at 100℃ for 5 min, then cooled on ice. The derivatized peak corresponding to ALA was separated by ultra performance -101- 4892-3380-3674.1
Atty. Dkt. No.: 115872-2919 liquid chromatography (UPLC) in a Waters Acquity UPLC system. The fluorescence detector was set at 370 nm excitation and 460 nm emission. The solvent system was 0.2% formic acid in water and pure methanol with a flow rate of 0.3 mL/min in a 12 min run on a gradient for peak separation. Each sample was run in triplicate, and ALAS activities were expressed as pmoles/h/mg protein. [00307] Quantitation of Total Heme Content in Murine Hepatocytes [00308] Total heme content was determined in isolated hepatocytes as previously described in Sinclair, Peter R., Nadia Gorman, and Judith M. Jacobs.1999. Current Protocols in Toxicology 00, no.1: 8.3.1-8.3.7. 2 M oxalic acid (0.5 mL) was added to hepatocyte lysates (~10 µg total protein), which were heated at 100ºC for 30 min to remove the iron component of heme. Samples were loaded onto a 96-well black plate and fluorescence (400 nm excitation and 608 nm emission) was recorded by the Synergy™ H1 microplate reader. Blank sample values (i.e., unheated samples) were subtracted from sample values, and these values were compared against a standard curve generated by heme solutions ranging from 0 to 25 µM in 40% DMSO. Heme concentrations were expressed as nmoles/mg protein. [00309] Quantitation of CYP1A2 Hemoprotein Activity in Murine Hepatocytes [00310] CYP1A2 activity was determined in isolated hepatocytes using the P450-Glo™ CYP1A2 Assay kit, according to the manufacturer’s instructions. Protein concentrations were determined as described above. Hepatocyte lysate (12.5 µl, corresponding to ~50 µg total protein) was added to a 96-well black plate, along with an equal volume of 4X CYP reaction mixture. Following a 10 min room temperature incubation, 25 µl of 2X nicotinamide adenine dinucleotide phosphate (NADPH) was added into each well to initiate CYP enzymatic reactions, and mixtures were incubated for another 10 min at room temperature. Reconstituted luciferin detection reagent (50 µl) was gently mixed in, and after a final 20 min room temperature incubation, luminescence was determined using the Synergy™ H1 microplate reader. Relative CYP1A2 activities were calculated by subtracting luminescence of CYP negative fractions (included in the kit) from sample luminescence values and expressed as relative luminescence units (RLU)/mg protein. -102- 4892-3380-3674.1
Atty. Dkt. No.: 115872-2919 [00311] Immunohistochemical (IHC) Staining for Cre-positive Hepatocytes in Alas1/2KO mouse [00312] IHC staining and imaging was performed by the Comparative Pathology Lab at Icahn School of Medicine. 5 µm paraffin-embedded tissues were blocked using 5% normal goat serum in 1x Tris-buffered saline with Tween 20 (TBST) and incubated with rabbit anti-GFP antibody (1:1200 diluted in the blocking solution), which detects Cre expression, for 16 h at 4ºC. Rinsed sections were incubated at room temperature for 40 min with goat anti-rabbit-HRP secondary antibody (1:200 diluted in blocking buffer) and HRP was visualized using the ImmPACT DAB Substrate Kit. Slides were counterstained with Richard-Allan Scientific hematoxylin 1, dehydrated and mounted with Richard-Allan scientific cytoseal 60. Images were captured with an Olympus BX43 microscope and Olympus DP21 digital microscopy camera. [00313] Alas1KO Primary Cell Culture Model and Transfection with Synthetic siRNAs [00314] PBS-perfused organs (i.e., brains, pancreas, eyes, lungs) were isolated from various Alas1KO mouse lines with Alas1 knockout/knockdown in neurons, astrocytes, microglia, retinal pigmental cells, pancreatic duct cells, or alveolar cells. Tissues were sliced and enzymatically dissociated. Alas1KO cells were isolated using magnetic beads conjugated to anti-GFP antibodies, and Alas1KO primary neuron, astrocyte, microglia, retinal pigmental epithelium, pancreatic ductal cells, and alveolar cell cultures were established in appropriate culture media. 24 h after establishing cultures, each Alas1KO cell line was individually transfected with a panel of commercially available siRNAs (e.g., Malat1, Actb, Hspa8, etc., for CNS cell lines; Crabp1, Dct, Silv, etc., for retinal pigmental epithelium; Krt19 and Sox9 for pancreatic ductal cells; Duox1 and Cit for alveolar cells) for 48 h and knockdown efficiency of mRNA of target genes were compared to those in respective Alas1-normal control cell lines. Example 2. Heme biogenesis contributes positively to nuclear cleavage of primary miRNA transcripts [00315] The large majority of well-expressed miRNAs in human cells are generated by a canonical pathway (FIG.1). This involves an initial hairpin cleavage by the nuclear RNase III enzyme Drosha and its double strand RNA binding (dsRBD) cofactor DGCR8 (together known -103- 4892-3380-3674.1
Atty. Dkt. No.: 115872-2919 as the Microprocessor complex), followed by cleavage of the hairpin by the cytoplasmic RNase III enyzme Dicer. The resulting miRNA duplex is loaded into an Argonaute (Ago) protein, which following removal of the passenger strand, uses the single stranded miRNA to identify complementary targets for regulation. Endogenous miRNAs can regulates genes with ~7 nucleotide (nt) pairing to their 5' termini via all four Ago-class effector proteins. If the small RNA is loaded to Ago2, the sole catalytic member of the family, and the target has extensive complementarity, it can be cleaved ("sliced"). This is the molecular activity that underlies experimental RNA interference (RNAi), which can be harnessed by loading synthetic small interfering RNA (siRNA) duplexes into Ago proteins (FIG.1). Certain non-canonical substrates can also yield functional small RNAs in Ago complexes (FIG.1). [00316] Heme is an essential cofactor for hemoglobin and numerous other cellular proteins. Heme is generated by a series of eight enzymes, for which the first step is mediated by ALAS1, which converts glycine and succinyl-CoA into δ-aminolevulinic acid (FIG.2). DGCR8 is a hemoprotein, and binding of hemin to its N-terminal Rhed domain stabilizes a DGCR8 dimer with the RNase III enzyme Drosha. This enhances the specificity and activity of primary miRNA (pri-miRNA) substrate cleavage (FIG.3). However, studies of hemin-binding by DGCR8 have mostly focused on in vitro tests of Microprocessor cleavage, or mutations of DGCR8 in cell-based assays. The impact of depleting heme biosynthetic factors on miRNA biogenesis in cells has not been addressed. [00317] To test whether heme biogenesis affects miRNA biogenesis in vivo, we mutated two core members of the heme biosynthetic pathway (ALAS1 and CPOX) in HEK293T cells, creating both single and double knockout lines. ALAS1 is broadly expressed across human tissues, while its functional paralog ALAS2 is restricted to erythroid settings (FIG.4). Importantly, knockout of ALAS1 does not result in compensation by ALAS2 in HEK293T cells (FIGs.5A-5B). Although these factors are essential for heme biogenesis, ALAS1 (the first enzyme in the 8-step biosynthetic pathway) is subject to feedback control, such that mutation of any downstream factor in the pathway results in upregulation of ALAS1. Accordingly, Western blotting -104- 4892-3380-3674.1
Atty. Dkt. No.: 115872-2919 confirmed that these mutations eliminated the corresponding proteins, but CPOX-KO also strongly upregulated ALAS1 protein (FIG.5C). [00318] With these novel validated cell reagents, we assessed the effects of knocking out core heme biogenesis factors on nuclear miRNA biogenesis. We generated a miRNA processing sensor in which pri-mir-375 is placed in the 3' UTR of a luciferase construct (FIG.6A). In principle, compromising Microprocessor activity should increase luciferase activity. However, no change was observed in either ALAS1 or CPOX knockouts (FIG.6B). Furthermore, we tested the accumulation of endogenous pri-miRNA transcripts in ALAS1 knockouts, and these also did not increase (FIG.6C), as would be expected if Microprocessor activity was defective. However, conventional fetal bovine serum contains high levels of heme (1-2 µM), which is sufficient to support cellular hemoproteins. Upon culturing ALAS1 or CPOX knockout cells with heme-depleted FBS, their health declined concomitant with increased luciferase activity from the miRNA sensor (FIG.6B). These data are consistent with a known role for heme for optimal Microprocessor function. This role is similar for core members of the heme biosynthetic pathway, such as ALAS1 and CPOX, but is obscured when culturing cells under conventional conditions. Example 3. ALAS1 represses miRNA accumulation in heme-replete conditions [00319] Despite the apparent lack of effect of removing core heme biosynthesis factors on nuclear miRNA processing under heme replete conditions, we analyzed these heme pathway mutants for effects on mature miRNA accumulation. Surprisingly, Northern blotting of RNAs from independent HEK293T ALAS1 knockout cell lines showed increased levels of mature miRNAs in total RNA samples (FIG.7A). These tests are internally controlled by blotting for 5S rRNA, which did not change in these tests reflecting equal loading. Functional miRNAs are carried within Argonaute complexes and guide them to targets for repression. We further validated that miRNA levels in immunoprecipitated Ago2 proteins are also enhanced in independent ALAS1 knockout cell lines (FIG.7B), revealing increased steady state presence of mature RISC in ALAS1 mutant cells. -105- 4892-3380-3674.1
Atty. Dkt. No.: 115872-2919 [00320] To evaluate broader impacts, we prepared small RNA sequencing libraries from control and ALAS1 knockout HEK293T cells. We anticipated a potentially broad and directional effect on miRNA expression, which might be confounded if using the typical strategy of normalizing datasets by library depth. Therefore, we used external spike-in controls for normalizing these libraries. Indeed, we observed a nearly universal increase in mature miRNA levels in ALAS1 knockout cells (FIG.8). Altogether these data establish, for the first time, that ALAS1 is an endogenous inhibitor of miRNA accumulation in vivo. Importantly, this novel activity is opposite to the expected role of heme as a positive factor for miRNA biogenesis, and suggested the existence of an unknown regulatory mechanism for miRNA biogenesis. Example 4. ALAS1 represses assembly of the RNA induced silencing complex (RISC) [00321] Because we did not observe substantial changes in pri-miRNA processing (FIG.6), or of pre-miRNA accumulation in heme-replete conditions (FIG.7A, e.g., pre-let-7) in ALAS1 knockout cells, we hypothesized they may impact at a late stage of miRNA biogenesis, following RNase III-mediated cleavages (FIG.1). We tested for a potential impact on terminal structures of miRNAs, which can affect miRNA accumulation. However, ALAS1 knockout did not impact 5'-monophosphates or 3'-hydroxyl groups on miRNA termini. This was determined by chemical treatments of small RNAs with phosphatase (CIP) or ß-elimination of 3' termini, which alter the gel migration of small RNAs. However, similar patterns of mobility shifts were observed with miRNAs isolated from ALAS1 knockout cells (FIG.9). [00322] We next examined potential alteration in formation of the RNA-induced silencing complex (RISC). Dicer-cleaved miRNA duplexes (and synthetic siRNA duplexes are loaded into Ago proteins, followed by removal of the passenger strand to yield a single-stranded miRNA guide in RISC. This can be monitored by assembling RISC in vitro with a miRNA duplex bearing a radiolabelled guide, and separating the products on native gels (FIG.10A). We find that the dynamics of RISC maturation are increased with lysates from independent Alas1- KO cell lines (FIGs.10B-10C). If RISC levels are increased in cells, we may expect concomitant changes in the association of mature Ago2 that associates with the essential cofactor GW182 (TNRC6), which mediates multiple downstream regulatory effects of Ago-miRNA -106- 4892-3380-3674.1
Atty. Dkt. No.: 115872-2919 silencing complexes (FIG.11A). Indeed, we reproducibly observed increased levels of Ago2 associated with GW182 in Alas1-KO cells (FIG.11B). Taken together, these tests establish a novel mechanistic basis by which removal of ALAS1 enhances the accumulation of mature miRNAs and RISC. Example 5. ALAS1 represses the miRNA pathway independently of other Heme biosynthetic factors [00323] Because our data unexpectedly pointed to ALAS1 as a repressor of the miRNA pathway, opposite to the known role for heme as a positive factor for efficient miRNA biogenesis, we conducted additional tests on the role of other core heme biosynthetic factors on the miRNA pathway in heme replete conditions. We first utilized our CPOX and CPOX/ALAS1 knockout cells (FIG.5). Although CPOX knockout induces ALAS1 protein (FIG.5C), all of these cells are equivalently incapable of cellular heme biosynthesis. However, CPOX knockout did not increase miRNA accumulation in heme replete conditions, and CPOX/ALAS1 double knockout exhibited similar effects as ALAS1 knockout (FIG.12A). In fact, the CPOX knockout condition reduced miRNA activity on a synthetic luciferase sensor, the opposite to the increase of miRNA activity in ALAS1 knockout and CPOX/ALAS1 double knockout cells (FIG.12B). This is consistent with the increased level of ALAS1 protein in CPOX knockout cells (FIG.5C), and our notion of ALAS1-dependent repression of the miRNA pathway. [00324] We also tested this panel of mutants in RISC assembly assays (FIG.10A). These analyses showed that CPOX knockout slightly reduced the efficiency of RISC formation, while CPOX/ALAS1 double knockout showed similar increase in RISC assembly as ALAS1 single knockout (FIGs.13A-13B). These data provide further evidence that ALAS1 represses miRNA biogenesis independently of the heme pathway per se. [00325] To enable more systematic tests of the novel role of ALAS1 in late stages of miRNA biogenesis, we utilized our synthetic pathway for RNase III-independent miRNA biogenesis, which uses hairpin cleavage by the tRNA enzyme RNaseZ to bypass Drosha, and direct loading of short mir-451 hairpins into Ago2 to bypass Dicer (FIG.14). We used this to generate a novel HEK293T cell line stably expressing tRNA-mir-451 and an RFP sensor bearing four miR-451 -107- 4892-3380-3674.1
Atty. Dkt. No.: 115872-2919 antisense matches (FIG.15A). Since miR-451 is not expressed in HEK293T cells, these specialized cell platform permits miRNA biogenesis and activity to be assayed, specifically downstream of Drosha and Dicer cleavage. In particular, depletion of factors such as ALAS1 are expected to result in enhanced silencing of miR-451 reporter, which could be read out with a single cell resolution using fluorescence activated cell sorting (FACS) (FIG.15B). [00326] We sought to mutate the tRNA-mir-451/miR-451 sensor recipient cell line by introducing Cas9 and sgRNAs against various heme pathway factors. These yield heterogenous mutations, as opposed to stable single-cell cloned mutant cells used for detailed functional characterization (FIG.5). We therefore validated their effects on ALAS1 protein, taking advantage of its feedback regulation. Western blotting showed ALAS1 sgRNAs clearly reduced ALAS1 protein, but the limitation of heterogeneous CRISPR/Cas9 editing was evident since these did not induce null conditions (FIG.16A). Conversely, we could infer the efficacy of PBGD, UROS, UROD, CPOX and FECH gRNAs, since all of them increased ALAS1 protein due to feedback regulation (FIG.16A). Therefore, these bulk cell assays indicate that heme pathway mutants can be induced by transient engineering of HEK293T cells with the CRISPR/Cas9 system, although the frequency of null cells might be variable. By assessing miRNA reporter activity in single cells with FACS, we can isolate the functional effects on null cells. These results clearly show that ALAS1 editing specifically yielded a population of cells with enhanced reporter silencing, but editing of the 5 other core heme biosynthetic factors did not (FIG.16B), despite clear functional validation of their efficient mutagenesis as measured by alteration of ALAS1 protein levels (FIG.16A). [00327] Altogether, these data confirm an unexpected and general role for ALAS1 in repressing the miRNA pathway, independently of other core heme biosynthesis factors. These mechanistic tests demonstrate that the repressive effect of ALAS lies downstream of RNase III- mediated cleavages, and impinges on the formation of the active RISC complex that mediates gene silencing. -108- 4892-3380-3674.1
Atty. Dkt. No.: 115872-2919 Example 6. Loss of ALAS1 increases occupancy of Ago-miRNA complexes across the transcriptome [00328] To extend from the study of individual synthetic miRNA targets (FIG.12) to the endogenous transcriptome, we performed Ago2 CLEAR-CLIP studies. In this procedure, ternary Ago2-miRNA-target complexes are UV crosslinked, immunopurified, treated with RNase I to refine mRNA footprints of Ago2 silencing complexes, and treated with ligase to obtain target mRNA-miRNA chimera (FIG.17A). The ligation step is low efficiency despite optimization; however, these chimeric reads provide valuable information since they directly identify individual miRNAs that are bound to target mRNAs inside active RISC. A key quality control in the generation of these complex libraries is the visualization of discrete, radiolabeled Ago2 complexes bearing miRNAs and target mRNA fragments (FIG.17B), which were used for library preparation. [00329] Analysis of the mapped Ago2 CLEAR-CLIP data at individual loci revealed that mRNA target fragments bearing seed matches to abundant HEK293T miRNAs, as well as direct chimera of such miRNAs with target site fragments, were elevated in ALAS1 knockout cells (FIG.18A, SERTAD3 bound by miR-92-3p). We independently validated that binding of Ago2 to SERTAD3 was elevated in ALAS1 knockout cells (FIG.18B). Furthermore, miRNA- dependent repression of the SERTAD33' UTR was elevated in ALAS1 knockout cells (FIG. 18C). We extended these findings to the transcriptome-wide level. First, the levels of miRNA- target chimera were increased in ALAS1 knockout cells (FIG.19A), reflecting enhanced engagement of Ago2/miRNA/mRNA ternary complexes. Second, miRNA-target chimera were enhanced across many different miRNAs, indicating that this is a general and directional effect, and was not biased by a minority of highly-abundant miRNAs (FIG.19B). Third, the levels of Ago2 binding to individual target loci was broadly increased across the transcriptome of ALAS1 knockout cells (FIG.19C). Together, these data demonstrate that ALAS1 broadly represses the accumulation of functionally engaged RISC within living cells. -109- 4892-3380-3674.1
Atty. Dkt. No.: 115872-2919 Example 7. Generation and characterization of inducible conditional hepatocyte-specific ALAS knockout mice [00330] To establish a mammalian model that permits knockdown of Alas1 in a temporal and tissue-specific manner, we generated a mouse line in which exons 4 and 5 of the murine Alas1 gene were flanked by tandemly oriented loxP sites using homologous recombination (Alas1- LoxP mice; FIGs.20A-20D). Alas1 exons 4 and 5 are 383 nucleotides combined, and therefore, excision of these two exons results in a frameshift and premature termination codon that occurs 8 nucleotides downstream of the exon 3/6 junction. [00331] Since givosiran and all other FDA-approved RNAi therapeutics (patisiran, inclisiran, lumasiran, vitrisiran) target hepatocyte genes, we initially generated inducible hepatocyte- specific ALAS1 knockout mice by crossing the above mouse line with Alb-Cre-ERT2 mice (Schuler M, Dierich A, Chambon P, Metzger D. Genesis.2004;39(3):167-172), which express Cre recombinase (Cre) under the control of the serum albumin promoter when induced with tamoxifen (TMX). Subsequently, they were bred against the well-characterized mT/mG reporter mice (Muzumdar MD, Tasic B, Miyamichi K, Li L, Luo L. Genesis.2007;45(9):593-605), which ubiquitously express red fluorescent tdTomato (mT) but only green fluorescence protein (EGFP, mG) in Cre-positive cells. The resulting C57BL/6 Alas1-LoxP
+/+/Cre-ERT2
+/-/mT/mG
+/- mice (designated iAlas1 mice) readily permit identification and enrichment of Cre-positive, Alas1- deficient hepatocytes. [00332] We induced male and female iAlas1 mice with an optimized TMX regimen (0.5 mg/day/mouse x 3 consecutive days, i.p.) to generate Alas1KO mice, which had liver specific excision of Alas1 exons 4 and 5 (FIG.21A). This resulted in >99% reduction of wildtype hepatic Alas1 mRNA compared to Cre-negative (Cre-) controls (i.e., Alas1-LoxP
+/+/Cre-ERT2
-/- mice administered equivalent TMX dose) and undetectable ALAS1 protein on Western blots (FIG.21B). Thus, we validate efficient, controlled knockout of Alas1 in the animal. [00333] Surprisingly and unexpectedly, we found that ‘erythroid-specific’ Alas2 mRNA is abundantly expressed in Alas1KO and Cre- control male mouse livers, whereas female mice have very little hepatic Alas2 expression (FIG.21C). Therefore, a siRNA targeting murine Alas2 -110- 4892-3380-3674.1
Atty. Dkt. No.: 115872-2919 (siAlas2) was generated and conjugated to N-acetylgalactosamine (GalNAc) for efficient and nearly-exclusive uptake by hepatocytes (Rajeev KG, Nair JK, Jayaraman M, et al. Chembiochem.2015;16(6):903-908; Springer AD, Dowdy SF. Nucleic Acid Ther. 2018;28(3):109-118). Alas1KO male and female mice administered a single dose of siAlas2 (10 mg/kg, s.c.) on the final day of TMX induction (resulting in Alas1/2KO mice) had >98% reduction of hepatic Alas1 and Alas2 mRNA (data not shown) and undetectable ALAS activity 3 weeks post- Alas1/2 ablation (FIG.21D). We confirmed that Cre expression is abundant and limited to hepatocytes and not expressed in other liver cells by immunohistochemistry (FIG. 21E). Example 8. ALAS depletion in hepatocytes is well-tolerated in vivo [00334] Despite having no discernable hepatic ALAS activity, Alas1/2KO male and female mice were clinically healthy and had normal complete blood counts, serum chemistry, and liver histology by H&E and Prussian blue stains (data not shown). It should be emphasized that Alas2 expression being present in hepatocytes is unique to mice. Human male and female hepatocytes do not express meaningful levels of ALAS2 mRNA. [00335] Moreover, we observe that Alas1/2-KO hepatocytes maintain near-normal heme content and activities of representative hemoproteins such as cytochrome P450 (CYP)1A2, nitric oxide synthase (NOS), and catalase (FIG.22), despite having markedly deficient Alas1 and Alas2 mRNA expression. Therefore, despite the fact that Alas genes are essential for heme biosynthesis, their validated conditional knockout in hepatocytes is tolerated well. We infer that Alas1/2-KO cells are able to obtain heme (and/or heme precursors) from extracellular sources, as shown in our ALAS1-KO cell culture model (FIG.6). These findings further support that it is the ALAS suppression itself, rather than decreased heme content, that leads to increased microRNAs. Example 9. ALAS depletion leads to increased miRNAs in vivo [00336] To assess whether depletion of ALAS in hepatocytes leads to increased miRNAs in vivo, male Alas1/2-KO and Cre- control mice were generated as described above and livers were harvested following digestion in situ using a two-step perfusion collagenase method (Nakatani T, -111- 4892-3380-3674.1
Atty. Dkt. No.: 115872-2919 Tsuboyama-Kasaoka N, Takahashi M, Miura S, Ezaki O. J Biol Chem.2002;277(11):9562- 9569). Liver cells were released into media and hepatocytes were isolated by centrifugation. We have established by fluorescence-activated cell sorting (FACS) that the liver cell population isolated using this method is highly enriched (>97%) for GFP-positive (i.e., Cre-positive) hepatocytes. [00337] We then used qPCR assays to confirm that Alas1/2-KO hepatocytes have >98% knockdown of Alas1 and Alas2 mRNAs compared to Cre- control hepatocytes, before subjecting samples to molecular characterization. [00338] Notably, we find that Alas1/2-KO hepatocytes exhibit global increases in mature miRNAs. Northern blotting of individual miRNAs confirms specific increase of multiple mature miRNAs, but not pre-miRNA intermediates (FIG.23A), supporting our proposal that ALAS represses the miRNA pathway downstream of RNase III cleavage. These results were consistent by quantification across multiple experiments (FIG.23B). Finally, we conducted global profiling by deep sequencing of small RNAs. We used spike-in controls to be able to normalize between library samples, which we hypothesized may exhibit globally biased miRNA levels. Indeed, we observe that Alas1/2-KO hepatocytes harbor global and directional increase in mature miRNAs (FIG.23C). [00339] We emphasize that despite the fact that ALAS activity is essential for intracellular heme biogenesis, and heme is an essential cofactor, validated Alas1/2-KO hepatocytes maintain near-normal heme content and activities of representative hemoproteins such as cytochrome P450 (CYP)1A2, nitric oxide synthase (NOS), and catalase (FIG.22). These findings strongly support the novel and unexpected finding that it is loss of ALAS per se, rather than decreased heme content or effects through other heme biosynthetic factors, that leads to increased miRNA levels. Example 10. ALAS1 mutation enhances experimental RNA silencing via miRNAs and siRNAs. [00340] Our knowledge of regulatory inhibition of miRNA mediated silencing leads us to propose that depletion of ALAS1 is a strategy to enhance experimental RNA silencing, either by -112- 4892-3380-3674.1
Atty. Dkt. No.: 115872-2919 miRNAs or chemically synthesized siRNAs. To test this concept, we performed two tests in our ALAS1-KO HEK293T cell system. First, we overexpressed our RNase III-independent tRNA- mir-451 construct, and evaluated its capacity to repress a trans-encoded luciferase reporter bearing four miR-451 binding sites (FIG.24A). We used this non-canonical miRNA system to separate out any potential effects on Drosha cleavage, pre-miRNA export, or Dicer cleavage, in ALAS1-KO cells. Indeed, we observed that independent ALAS1-KO cells reproducibly supported increased silencing of the miR-451 sensor (FIG.24B). [00341] Since we were interested to expand the scope of this concept to synthetic siRNAs, which are used for experimental and therapeutic RNA silencing, we set up a GFP transgene system in control and ALAS1-KO HEK293T cells. These stable GFP-expressing lines were selected to have equivalent fluorescence, to enable head-to-head comparison. Treatment of these lines with chemically synthesized siRNA duplex against GFP coding sequence (siGFP) is expected to reduce GFP protein levels (FIG.24C). Indeed, 24 hours after introduction of siGFP to cells, we observed stronger suppression of GFP protein in ALAS1-KO cells compared to parental wildtype cells (FIG.24D), and this effect was observed reproducibly (FIG.24E). This proof-of-principle tests support our concept that ALAS1 deficiency is a suitable genetic background for enhanced small RNA-mediated silencing. Example 11. ALAS suppression leads to enhanced siRNA-mediated silencing of specific hepatocyte gene targets in vivo [00342] To evaluate whether ablation of hepatocyte ALAS expression enhances siRNA- mediated silencing of specific hepatocyte gene targets in vivo, we administer into Alas1/2KO male mice and age-and sex-matched Cre-control mice a GalNAc-conjugated siRNA targeting a gene other than Alas1 or Alas2 (e.g., Slc46A1). We showed that knockdown of the non-Alas- siRNA target (e.g., Slc46A1) is enhanced in the Alas1/2KO males compared to sex- and age- matched Cre- control mice administered the equivalent dose of the non-Alas-siRNA (e.g., Slc46A1-targeted siRNA) (FIG.25). -113- 4892-3380-3674.1
Atty. Dkt. No.: 115872-2919 Example 12. Givosiran enhances siRNA-mediated silencing of specific hepatocyte gene targets in vivo [00343] We then will test whether suppression of hepatocyte Alas1 via administration of givosiran effectively enhances siRNA-mediated silencing of specific hepatocyte gene targets in wildtype female mice. Givosiran comprises an antisense strand including the sequence AUGAGACACUCUUUCUGGU (SEQ ID NO: 95) and the sense strand including the sequence ACCAGAAAGAGUGUCUCAU (SEQ ID NO: 96). [00344] 48 h following administration of a single dose of givosiran (2.5 mg/kg; s.c.), wildtype female mice are administered a single dose of a different GalNAc-conjugated siRNA targeting a gene other than Alas1 or Alas2 (e.g., GalNAc-conjugated siRNA targeting hepatocyte hemopexin, ferrochelatase, or Slc46A1). Age-matched control wildtype females are administered an equivalent dose of the non-Alas-siRNA target (e.g., hemopexin, ferrochelatase, or Slc46A1) but are not pre-treated with givosiran. Administration doses of the Fech, Hpx, or Slc46A1-targeted siRNAs will be optimized so that they knock down target gene expression by 25-50% in hepatocytes in the absence of ALAS suppression/depletion. [00345] It is anticipated that silencing of the non-Alas-siRNA target will be enhanced in the presence of givosiran. Together, these experiments demonstrate that suppression of hepatocyte ALAS activity enhances the effects of synthetic siRNAs in vivo and support the use of givosiran as an RNAi adjuvant in hepatocytes. While there are currently four approved hepatocyte- directed siRNA therapies excluding givosiran (patisiran, lumasiran, vutrisiran, inclisiran), many more are in clinical development, including cemdisiran (for paroxysmal nocturnal hemoglobinuria), zilebesiran (for hypertension), ALN-XDH (for gout), ALN-HSD (for nonalcoholic steatohepatitis), and AB-729 (for hepatitis B). Example 13. ALAS depletion leads to enhanced siRNA-mediated silencing of synthetic siRNAs in various extra-hepatocytic cell types including neurons (CNS), astrocytes (CNS), -114- 4892-3380-3674.1
Atty. Dkt. No.: 115872-2919 and microglia (CNS), retinal pigmental epithelium (eye), pancreatic ductal cells, and alveolar cells (lungs) [00346] To evaluate whether cell types other than hepatocytes enhance RNAi efficiency in response to Alas1 suppression/depletion, we are generating additional TMX-inducible mouse lines that permit Alas1 knockout specifically in neurons, astrocytes, microglia, retinal pigmental epithelium, pancreatic ductal cells, or alveolar cells. This is important as siRNA therapeutics targeting extra-hepatic tissues, including the central nervous system are being developed (Brown KM, Nair JK, Janas MM, et al. Nat Biotechnol.2022;40(10):1500-1508). In fact, Alnylam is performing Phase I clinical trials evaluating ALN-APP, a C16-conjugated siRNA against amyloid precursor protein for the treatment of early-onset Alzheimer’s disease and cerebral amyloid angiopathy. C16-conjugated siRNAs were found to be effectively targeted not only to cells of the CNS (neurons, astrocytes, microglial cells), but also the eye (retinal pigmental epithelium) and lungs (alveolar and bronchial cells). A number of other biotech companies (Quark, Loxo@Lilly, Amgen, etc.) are also performing Clinical Trials for siRNA therapeutics for various non-hepatic cancers (e.g., pancreatic ductal adenocarcinoma, relapsed or refractory B cell non-Hodgkin lymphoma, etc.), eye disorders (e.g., dry eye disease, open angle glaucoma, etc.) and acute kidney injury. [00347] Initial efforts will evaluate the in vivo safety of Alas1 suppression/depletion in the above listed cell types (i.e., CNS neurons, astrocytes, and microglia, and retinal pigmental epithelium, pancreatic ductal cells, and alveolar cells). Following TMX-induced Alas1 suppression/depletion in the various mouse lines, it is anticipated that daily monitoring of the mice for up to a month will show normal general appearance, activity levels, and body weights, supporting the safety of Alas1 suppression/depletion in extra-hepatocytic cells. [00348] In vitro Alas1KO neuron, astrocyte, microglial, retinal pigmental epithelium, pancreatic ductal cells, or alveolar cell culture models from the respective TMX-induced Alas1 mouse lines will be established. It is anticipated that when the Alas1KO cell lines are individually incubated with a panel of siRNAs targeting various genes, silencing of the siRNA -115- 4892-3380-3674.1
Atty. Dkt. No.: 115872-2919 target will be enhanced compared to their respective Alas1-intact control cultures. These findings demonstrate the generality of Alas1 suppression to enhance RNAi. EQUIVALENTS [00349] The present technology is not to be limited in terms of the particular embodiments described in this application, which are intended as single illustrations of individual aspects of the present technology. Many modifications and variations of this present technology can be made without departing from its spirit and scope, as will be apparent to those skilled in the art. Functionally equivalent methods and apparatuses within the scope of the present technology, in addition to those enumerated herein, will be apparent to those skilled in the art from the foregoing descriptions. Such modifications and variations are intended to fall within the scope of the present technology. It is to be understood that this present technology is not limited to particular methods, reagents, compounds compositions or biological systems, which can, of course, vary. It is also to be understood that the terminology used herein is for the purpose of describing particular embodiments only, and is not intended to be limiting. [00350] In addition, where features or aspects of the disclosure are described in terms of Markush groups, those skilled in the art will recognize that the disclosure is also thereby described in terms of any individual member or subgroup of members of the Markush group. [00351] As will be understood by one skilled in the art, for any and all purposes, particularly in terms of providing a written description, all ranges disclosed herein also encompass any and all possible subranges and combinations of subranges thereof. Any listed range can be easily recognized as sufficiently describing and enabling the same range being broken down into at least equal halves, thirds, quarters, fifths, tenths, etc. As a non-limiting example, each range discussed herein can be readily broken down into a lower third, middle third and upper third, etc. As will also be understood by one skilled in the art all language such as “up to,” “at least,” “greater than,” “less than,” and the like, include the number recited and refer to ranges which can be subsequently broken down into subranges as discussed above. Finally, as will be understood by one skilled in the art, a range includes each individual member. Thus, for example, a group -116- 4892-3380-3674.1
Atty. Dkt. No.: 115872-2919 having 1-3 cells refers to groups having 1, 2, or 3 cells. Similarly, a group having 1-5 cells refers to groups having 1, 2, 3, 4, or 5 cells, and so forth. [00352] All patents, patent applications, provisional applications, and publications referred to or cited herein are incorporated by reference in their entirety, including all figures and tables, to the extent they are not inconsistent with the explicit teachings of this specification. -117- 4892-3380-3674.1