We derive a variational cluster approximation for Heisenberg spin systems at finite temperature based on the ideas of the self-energy functional theory by Potthoff for fermionic and bosonic systems with local interactions. Partitioning the real system into a set of clusters, we find an analytical expression for the auxiliary free energy, depending on a set of variational parameters defined on the cluster, whose stationary points provide approximate solutions from which the thermodynamics of spin models can be obtained. We explicitly describe the technical details of how to evaluate the free energy for finite clusters and remark on specific problems and possible limitations of the method. To test the approximation we apply it to the antiferromagnetic spin chain and compare the results for varying cluster sizes and choices of variational parameters with the exact Bethe ansatz solution.
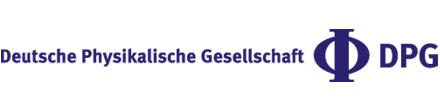
The Deutsche Physikalische Gesellschaft (DPG) with a tradition extending back to 1845 is the largest physical society in the world with more than 61,000 members. The DPG sees itself as the forum and mouthpiece for physics and is a non-profit organisation that does not pursue financial interests. It supports the sharing of ideas and thoughts within the scientific community, fosters physics teaching and would also like to open a window to physics for all those with a healthy curiosity.
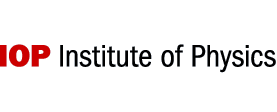
The Institute of Physics (IOP) is a leading scientific society promoting physics and bringing physicists together for the benefit of all. It has a worldwide membership of around 50 000 comprising physicists from all sectors, as well as those with an interest in physics. It works to advance physics research, application and education; and engages with policy makers and the public to develop awareness and understanding of physics. Its publishing company, IOP Publishing, is a world leader in professional scientific communications.
ISSN: 1367-2630
New Journal of Physics (NJP) publishes important new research of the highest scientific quality with significance across a broad readership. The journal is owned and run by scientific societies, with the selection of content and the peer review managed by a prestigious international board of scientists.
S Filor and T Pruschke 2014 New J. Phys. 16 063059
Ran Finkelstein et al 2023 New J. Phys. 25 035001
This tutorial introduces the theoretical and experimental basics of electromagnetically induced transparency (EIT) in thermal alkali vapors. We first give a brief phenomenological description of EIT in simple three-level systems of stationary atoms and derive analytical expressions for optical absorption and dispersion under EIT conditions. Then we focus on how the thermal motion of atoms affects various parameters of the EIT system. Specifically, we analyze the Doppler broadening of optical transitions, ballistic versus diffusive atomic motion in a limited-volume interaction region, and collisional depopulation and decoherence. Finally, we discuss the common trade-offs important for optimizing an EIT experiment and give a brief 'walk-through' of a typical EIT experimental setup. We conclude with a brief overview of current and potential EIT applications.
Florian Metzler et al 2024 New J. Phys. 26 101202
We investigate known mechanisms for enhancing nuclear fusion rates at ambient temperatures and pressures in solid-state environments. In deuterium fusion, on which the paper is focused, an enhancement of >40 orders of magnitude would be needed to achieve observable fusion. We find that different mechanisms for fusion rate enhancement are known across the domains of atomic physics, nuclear physics, and quantum dynamics. Cascading multiple such mechanisms could lead to an overall enhancement of 40 orders of magnitude or more. We present a roadmap with examples of how hypothesis-driven research could be conducted in—and across—each domain to probe the plausibility of technologically-relevant fusion in the solid state.
Joaquín J Torres and Daniel Manzano 2024 New J. Phys. 26 103018
We present extensive simulations of a quantum version of the Hopfield neural network to explore its emergent behavior. The system is a network of N qubits oscillating at a given Ω frequency and which are coupled via Lindblad jump operators built with local fields hi depending on some given stored patterns. Our simulations show the emergence of pattern-antipattern oscillations of the overlaps with the stored patterns similar (for large Ω and small temperature) to those reported within a recent mean-field description of such a system, and which are originated deterministically by the quantum term including qubit operators. However, in simulations we observe that such oscillations are stochastic due to the interplay between noise and the inherent metastability of the pattern attractors induced by quantum oscillations, and then are damped in finite systems when one averages over many quantum trajectories. In addition, we report the system behavior for large number of stored patterns at the lowest temperature we can reach in simulations (namely
). Our study reveals that for small-size systems the quantum term of the Hamiltonian has a negative effect on storage capacity, decreasing the overlap with the starting memory pattern for increased values of Ω and number of stored patterns. However, it also impedes the system to be trapped for long time in mixtures and spin-glass states. Interestingly, the system also presents a range of Ω values for which, although the initial pattern is destabilized due to quantum oscillations, other patterns can be retrieved and remain stable even for many stored patterns, implying a quantum-dependent nonlinear relationship between the recall process and the number of stored patterns.
Roger Bach et al 2013 New J. Phys. 15 033018
Double-slit diffraction is a corner stone of quantum mechanics. It illustrates key features of quantum mechanics: interference and the particle-wave duality of matter. In 1965, Richard Feynman presented a thought experiment to show these features. Here we demonstrate the full realization of his famous thought experiment. By placing a movable mask in front of a double-slit to control the transmission through the individual slits, probability distributions for single- and double-slit arrangements were observed. Also, by recording single electron detection events diffracting through a double-slit, a diffraction pattern was built up from individual events.
Antonio Acín et al 2018 New J. Phys. 20 080201
Within the last two decades, quantum technologies (QT) have made tremendous progress, moving from Nobel Prize award-winning experiments on quantum physics (1997: Chu, Cohen-Tanoudji, Phillips; 2001: Cornell, Ketterle, Wieman; 2005: Hall, Hänsch-, Glauber; 2012: Haroche, Wineland) into a cross-disciplinary field of applied research. Technologies are being developed now that explicitly address individual quantum states and make use of the 'strange' quantum properties, such as superposition and entanglement. The field comprises four domains: quantum communication, where individual or entangled photons are used to transmit data in a provably secure way; quantum simulation, where well-controlled quantum systems are used to reproduce the behaviour of other, less accessible quantum systems; quantum computation, which employs quantum effects to dramatically speed up certain calculations, such as number factoring; and quantum sensing and metrology, where the high sensitivity of coherent quantum systems to external perturbations is exploited to enhance the performance of measurements of physical quantities. In Europe, the QT community has profited from several EC funded coordination projects, which, among other things, have coordinated the creation of a 150-page QT Roadmap (http://qurope.eu/h2020/qtflagship/roadmap2016). This article presents an updated summary of this roadmap.

L M Hoyos-Campo et al 2024 New J. Phys. 26 103033
Results of spectroscopy experiments using the electric dipole forbidden transition in cold rubidium atoms are presented. Production of this forbidden transition is detected by observing the emission of the 420 nm fluorescence photons that result from the decay of the
state into the ground state. The experiments are performed under the steady state operation conditions of a magneto-optical trap (MOT), and thus provide non-perturbing information on the atom-light system. The hyperfine structure of the
level is completely resolved, and the fluorescence peaks show the expected Autler–Townes (AT) splitting of the emission lines. This hyperfine structure is used to calibrate the frequency scale of all spectra recorded. A combination of this absolute frequency scale and an expression for the AT profile allows an absolute determination of the effective Rabi frequency and detuning of the MOT. The behavior of these AT doublets is studied as a function of frequency detuning and also as a function of the trapping light intensity. The experiments also take full advantage of the strong polarization dependence of the relative intensities of the hyperfine fluorescence components. This study results in a sensitive probe of the relative populations of the magnetic sublevel projections relative to the trapping magnetic field gradient. An almost isotropic population distribution was found, but small deviations from isotropy could be determined with this electric dipole forbidden probe.
Caroline Cohen et al 2015 New J. Phys. 17 063001
The conical shape of a shuttlecock allows it to flip on impact. As a light and extended particle, it flies with a pure drag trajectory. We first study the flip phenomenon and the dynamics of the flight and then discuss the implications on the game. Lastly, a possible classification of different shots is proposed.
Anita Pahi et al 2024 New J. Phys. 26 103038
We report a study on the dynamics of absorbing asymmetric carbon clusters trapped by a loosely focused Gaussian beam using photophoretic force. At high laser powers, all the trapped clusters display rotation coupled with oscillation along the axial direction, with a majority spinning about a body fixed axis, while the rest display dual spin as well as orbital motion about a fixed point in space. The spinning and orbiting frequency is inversely proportional to the amplitude of the axial oscillation–with one growing at the expense of the other. Further, the frequencies of these rotations are not proportional to the laser power, but to the trap stiffnesses inferred from the corresponding natural frequencies. The clusters also stop rotating below a certain laser power, and execute random thermal fluctuations. Our work suggests that the dynamics of clusters trapped with photophoretic force are largely dependent on the cluster size and morphology, which could, in principle, be tuned to obtain various motional responses, and help in the design of rotating micromachines in air.
S Danilin et al 2024 New J. Phys. 26 103029
Sensing and metrology are crucial in both fundamental science and practical applications. They meet the constant demand for precise data, enabling more dependable assessments of theoretical models' validity. Sensors, now a common feature in many fields, play a vital role in applications like gravity imaging, geology, navigation, security, timekeeping, spectroscopy, chemistry, magnetometry, healthcare, and medicine. The advancements in quantum technologies have sparked interest in employing quantum systems as sensors, offering enhanced capabilities and new possibilities. This article describes the optimization of the quantum-enhanced sensing of magnetic fluxes with a Kitaev phase estimation algorithm based on frequency tunable transmon qubits. It provides the optimal flux biasing point for sensors with different qubit transition frequencies and gives an estimation of decoherence rates and achievable sensitivity. The use of 2- and 3-qubit entangled states are compared in simulation with the single-qubit case. The flux sensing accuracy reaches and scales inversely with time, which proves the speed-up of sensing with high ultimate accuracy.
Emil Lenler-Eriksen et al 2024 New J. Phys. 26 113008
In the last decade, a growing interest has been devoted to models of spontaneous collapse of the wavefunction, known also as collapse models. They coherently solve the well-known quantum measurement problem by suitably modifying the Schrödinger evolution. Quantum experiments are now finally within the reach of testing such models (and thus testing the limits of quantum theory). Here, we propose a method based on a two-ions confined in a linear Paul trap to possibly enhance the testing capabilities of such experiments. The combination of an atomic and a macromolecular ion provide a good match for the cooling of the motional degrees of freedom and a non-negligible insight in the collapse mechanism, respectively.
J C Bellizotti Souza et al 2024 New J. Phys. 26 113007
Using atomistic simulations, we investigate the dynamical behavior of magnetic skyrmions in dimer and trimer molecular crystal arrangements, as well as bipartite lattices at 3/2 and 5/2 fillings, under ac driving over a square array of anisotropy defects. For low ac amplitudes, at all fillings reversible motion appears in which the skyrmions return to their original positions at the end of each ac drive cycle and the diffusion is zero. We also identify two distinct irreversible regimes. The first is a translating regime in which the skyrmions form channels of flow in opposing directions and translate by one substrate lattice constant per ac drive cycle. The translating state appears in the dimer and trimer arrangements, and produces pronounced peaks in the diffusivity in the direction perpendicular to the external drive. For larger ac amplitudes, we find chaotic irreversible motion in which the skyrmions can randomly exchange places with each other over time, producing long-time diffusive behavior both parallel and perpendicular to the ac driving direction.
Guodong Yu et al 2024 New J. Phys. 26 113006
The symmetry of twisted bilayer graphene (tBG) has been extensively studied in the continuum model but somewhat overlooked in the tight-binding (TB) framework. In contrast to the continuum model, which requires operators for both sublattice and layer spaces, the TB framework relies solely on operators in real space. This paper begins by discussing the symmetries of the TB Hamiltonian and the single-valley TB Hamiltonian of tBG. Subsequently, the band structures with specific irreducible representations (irreps) are obtained by diagonalizing the irrep-dependent Hamiltonian constructed through projection operators. We also investigate the impact of symmetry on intervalley coupling and the influence of a non-zero mass term on the symmetry of the single-valley Hamiltonian. To understand the irrep change as the wavevector moves inside the Brillouin zone and the irrep change after considering the intervalley coupling or introducing a non-zero mass term at a fixed wavevector, we derive two kinds of compatibility relationships. The methodology presented here can be applied to other layered materials because the basis functions we employ are generic and unique to twisted bilayers.
Zhuoming Ren et al 2024 New J. Phys. 26 113005
World trade networks are exhaustively described by pairwise interactions, and overlook higher-order structure from the outcome of collective interactions at the level of groups of nodes like multilateral trade agreements. To address this limitation, we collect multiplex world trade networks, including the bilateral regional trade agreement network, which represents pairwise interactions; the multilateral regional trade agreement network, which naturally represents a higher-order network structure; and the import and export trade network, which represents pairwise interactions and additional complexities. The analysis of simplicial centrality, including degree, closeness, and subgraph at 0, 1, and 2-simplex levels, reveals that intra-level correlations are high, while inter-levels may exhibit significant disparities. Nodes with low centrality at higher-order levels could influence network robustness due to the diversity of interactions and higher-order dependencies. Simplicial centrality on robustness of multiplex world trade networks under random and targeted attacks reveals that the complex connectivity of higher-order levels renders them more vulnerable post-attack. An optimization strategy of the rebalancing of network centrality is proposed to enhance the robustness, and the simulation shows risks posed to central nodes are minimized and opportunities for peripheral nodes to partake in global trade are broadened.
Laria Figurato et al 2024 New J. Phys. 26 113004
The possibility that gravity plays a role in the collapse of the quantum wave function has been considered in the literature, and it is of relevance not only because it would provide a solution to the measurement problem in quantum theory, but also because it would give a new and unexpected twist to the search for a unified theory of quantum and gravitational phenomena, possibly overcoming the current impasse. The Diósi–Penrose model is the most popular incarnation of this idea. It predicts a progressive breakdown of quantum superpositions when the mass of the system increases; as such, it is susceptible to experimental verification. Current experiments set a lower bound Å for the free parameter of the model, excluding some versions of it. In this work we search for an upper bound, coming from the request that the collapse is effective enough to guarantee classicality at the macroscopic scale: we find out that not all macroscopic systems collapse effectively. If one relaxes this request, a reasonable (although to some degree arbitrary) bound is found to be:
Å. This will serve to better direct future experiments to further test the model.
Florian Metzler et al 2024 New J. Phys. 26 101202
We investigate known mechanisms for enhancing nuclear fusion rates at ambient temperatures and pressures in solid-state environments. In deuterium fusion, on which the paper is focused, an enhancement of >40 orders of magnitude would be needed to achieve observable fusion. We find that different mechanisms for fusion rate enhancement are known across the domains of atomic physics, nuclear physics, and quantum dynamics. Cascading multiple such mechanisms could lead to an overall enhancement of 40 orders of magnitude or more. We present a roadmap with examples of how hypothesis-driven research could be conducted in—and across—each domain to probe the plausibility of technologically-relevant fusion in the solid state.
Mingyu Lee et al 2024 New J. Phys. 26 101201
Alkali atomic vapor lasers have gained significant attention in recent decades as a promising option for high-powered and efficient laser systems. Utilizing hot alkali atomic vapor as the optical gain medium, these lasers, in principle, offer several advantages, such as high quantum efficiency, reduced thermal issues, and high beam quality. This paper reviews critical techniques developed in recent years to enhance the power and efficiency of these lasers. We discuss continuous wave laser optimization strategies, optical amplifier schemes, and pulsed laser generation based on hot alkali atomic vapor cells. Additionally, select findings from the authors' research group are presented.
Sharath S Girimaji 2024 New J. Phys. 26 071201
Turbulence closure modeling using (ML) is at an early crossroads. The extraordinary success of ML in a variety of challenging fields had given rise to an expectation of similar transformative advances in the area of turbulence closure modeling. However, by most accounts, the current rate of progress toward accurate and predictive ML-RANS (Reynolds Averaged Navier–Stokes) closure models has been very slow. Upon retrospection, the absence of rapid transformative progress can be attributed to two factors: the underestimation of the intricacies of turbulence modeling and the overestimation of ML's ability to capture all features without employing targeted strategies. To pave the way for more meaningful ML closures tailored to address the nuances of turbulence, this article seeks to review the foundational flow physics to assess the challenges in the context of data-driven approaches. Revisiting analogies with statistical mechanics and stochastic systems, the key physical complexities and mathematical limitations are explicated. It is noted that the current ML approaches do not systematically address the inherent limitations of a statistical approach or the inadequacies of the mathematical forms of closure expressions. The study underscores the drawbacks of supervised learning-based closures and stresses the importance of a more discerning ML modeling framework. As ML methods evolve (which is happening at a rapid pace) and our understanding of the turbulence phenomenon improves, the inferences expressed here should be suitably modified.
Xuan Zuo et al 2024 New J. Phys. 26 031201
Hybrid quantum systems based on magnons in magnetic materials have made significant progress in the past decade. They are built based on the couplings of magnons with microwave photons, optical photons, vibration phonons, and superconducting qubits. In particular, the interactions among magnons, microwave cavity photons, and vibration phonons form the system of cavity magnomechanics (CMM), which lies in the interdisciplinary field of cavity QED, magnonics, quantum optics, and quantum information. Here, we review the experimental and theoretical progress of this emerging field. We first introduce the underlying theories of the magnomechanical coupling, and then some representative classical phenomena that have been experimentally observed, including magnomechanically induced transparency, magnomechanical dynamical backaction, magnon-phonon cross-Kerr nonlinearity, etc. We also discuss a number of theoretical proposals, which show the potential of the CMM system for preparing different kinds of quantum states of magnons, phonons, and photons, and hybrid systems combining magnomechanics and optomechanics and relevant quantum protocols based on them. Finally, we summarize this review and provide an outlook for the future research directions in this field.
J Lambert and E S Sørensen 2023 New J. Phys. 25 081201
Recently, there has been considerable interest in the application of information geometry to quantum many body physics. This interest has been driven by three separate lines of research, which can all be understood as different facets of quantum information geometry. First, the study of topological phases of matter characterized by Chern number is rooted in the symplectic structure of the quantum state space, known in the physics literature as Berry curvature. Second, in the study of quantum phase transitions, the fidelity susceptibility has gained prominence as a universal probe of quantum criticality, even for systems that lack an obviously discernible order parameter. Finally, the study of quantum Fisher information in many body systems has seen a surge of interest due to its role as a witness of genuine multipartite entanglement and owing to its utility as a quantifier of quantum resources, in particular those useful in quantum sensing. Rather than a thorough review, our aim is to connect key results within a common conceptual framework that may serve as an introductory guide to the extensive breadth of applications, and deep mathematical roots, of quantum information geometry, with an intended audience of researchers in quantum many body and condensed matter physics.
Schlichtholz et al
Achieving resolution in the sub-Rayleigh regime (superresolution) is one of the rapidly developing topics in quantum optics and metrology. Recently, it was shown that perfect measurement based on spatial mode demultiplexing (SPADE) in Hermite-Gauss modes allows one to reach the quantum limit of precision for estimation of separation between two weak incoherent stationary sources. Since then, different imperfections such as misalignment or crosstalk between modes have been studied to check how this result translates into more realistic experimental setups. In this paper, we consider another deviation from the perfect setup by discarding the assumption about the stationarity of the sources. This is relevant for example for astrophysical applications where planets necessarily orbit around the star. We analyze two examples of dynamics: rotations and oscillations, showing the robustness of the SPADE-based measurement against them. The analysis is based on Fisher information, which allows one to obtain the precision limit through Cramér-Rao bound. Furthermore, we formulate a measurement algorithm that allows for the reduction of one parameter for estimation (system orientation angle) in the stationary sources scenario, maintaining the measurement precision despite the lack of knowledge about this parameter.
Zhao et al
The effect of an external magnetic field on the evolution of the self-generated electromagnetic field during laser ablation is investigated by using the Vlasov-Fokker-Planck simulations. It is found that the self-generated field is rotated and distorted under an external magnetic field, and for highly magnetized plasma, the rotation of the electric field becomes stable after the laser ablation. The theoretical analysis indicates that the rotation and tortuosity are primarily attributed to the advection of the Nernst effect and the Righi-Leduc (RL) flux. The curl of the self-generated field increases with the Hall parameter χe and reaches a peak at χe = 0.075, then it decreases with the χe continuous increase. As the Hall parameter increases, the RL flux contributes more than 60% to the rotation of the electric field. Furthermore, the distortion of the electric field continues to rotate after the laser ablation due to the cross-gradient Nernst transport. These findings provide theoretical references for the evolution of the self-generated electromagnetic field in laser-driven magnetized plasmas.
Li et al
Thermal nonreciprocity are typically realized by using nonlinear heat conductivity, external field bias or spatiotemporal modulation of thermal parameters, but these methods require specific material properties or accurate modulation means. Low-dimensional materials like graphene provides a platform to engineer heat transport characteristics at the molecular level. Here, by simply tuning graphene's wrinkle morphol ogy, the approach to generate nonreciprocal heat transfer is reported, which applies to both static and dynamic heat signals. Such nonreciprocity is attributed to the tunable responses of thermal conductivity on both temperatures and wrinkles. Phonon density of states (PDOS) of the wrinkled graphene exhibit distinct spectra, especially at the low frequency (around 17 THz), which results in reduced temperature sensitivity of thermal conductivities and eventually induces thermal nonreciprocity. The findings provide insights into the physics of thermal transport in wrinkled graphene, and paves a new avenue for mechan ically modulating thermal nonreciprocity. Our method to obtain thermal nonreciprocity is reversible, tunable and controllable, meanwhile remains structural integrity, which benefits functional applications such as heat management in electronics.
Cheng et al
We investigate the off-resonant circularly polarized light-modulated crossed Andreev reflection in an 8-Pmmn borophene-based normal conductor/superconductor/normal conductor junction. When the signs of Fermi energies in two normal regions are opposite, the pure crossed Andreev reflection without the local Andreev reflection and the elastic cotunneling occurs. By using the Dirac-Bogoliubov-de Gennes equation and the Blonder-Tinkham-Klapwijk formula, the pure crossed Andreev reflection conductance and its oscillation as a function of the junction length and the Fermi energy in the superconducting regions are discussed. It is found that the value of pure crossed Andreev reflection conductance peak value and its corresponding value of light-induced gap increase with the increase of incident energy of electron. Furthermore, the valley splitting for the transmitted hole is found due to the presence of tilted velocity of borophene. Our findings are beneficial for designing the high efficiency 8-Pmmn borophene-based nonlocal transistor and nonlocal valley splitter without local and non-entangled processes.
Wiesner et al
While the advantages of photonic quantum computing, including direct compatibility with communication, are apparent, several imperfections such as loss and distinguishability presently limit actual implementations. These imperfections are unlikely to be completely eliminated, and it is therefore beneficial to investigate which of these are the most dominant and what is achievable under their presence. In this work, we provide an in-depth investigation of the influence of photon loss, multi-photon terms and photon distinguishability on the generation of photonic 3-partite GHZ states via established fusion protocols. 
We simulate the generation process for SPDC and solid-state-based single-photon sources using realistic parameters and show that different types of imperfections are dominant with respect to the fidelity and generation success probability. 
Our results indicate what are the dominant imperfections for the different photon sources and in which parameter regimes we can hope to implement photonic quantum computing in the near future.