Chemicals/Berylliums

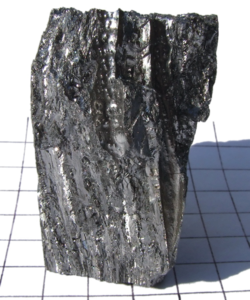
The "presence in ... cosmic radiation [is] of a much greater proportion of "secondary" nuclei, such as lithium, beryllium and boron, than is found generally in the universe."[1]
Bromellite is a mineral found on Earth composed of BeO, with 50 at % beryllium.[2]
Emissions
[edit | edit source]
The visual spectrum for beryllium is missing characteristic lines in the yellow and orange.
Beryllium has at least six emission/absorption lines across the red.
The emission and absorption spectra for beryllium contain lines in the blue.
Above is a light spectrum of the emission and absorption lines of neutral, atomic beryllium.
Particles
[edit | edit source]Notation: let the symbol DPM represent Diesel vehicle exhaust Particulate Matter.
"Moreover DPM can bear metals like antimony, arsenic, beryllium, cadmium, chromium, copper, iron, lead, magnesium, manganese, nickel, vanadium and zinc ... the dominant group of particles had a diameter of 20 to 25 nm (at 900 rpm 50% load or 25 to 30 nm 100% load) for individual soot nodules."[3]
Alpha particles
[edit | edit source]A photon carrying 1.67 MeV or more energy can photodisintegrate an atom of beryllium-9 (100% of natural beryllium, its only stable isotope):
- 9
4Be
(γ,n) 2α
Antimony-124 is assembled with beryllium to make laboratory neutron sources and startup neutron sources. Antimony-124 (half-life 60.20 days) emits β− and 1.690 MeV gamma rays (also 0.602 MeV and 9 fainter emissions from 0.645 to 2.090 MeV), yielding stable tellurium-124. Gamma rays from Antimony-124 knock neutrons off beryllium-9 with an average kinetic energy of 24keV, intermediate neutrons. The other product is two alpha particles.[4][5]
Other isotopes have higher thresholds for photoneutron production, as high as 18.72 MeV, for carbon-12.[6]
Cosmic rays
[edit | edit source]Def. "an energetic particle originating outside our solar system"[7] is called a cosmic ray.
"Cosmic rays arise from galactic source accelerators."[8]
Cosmic rays may be upwards of a ZeV (1021 eV).
About 89% of cosmic rays are simple protons or hydrogen nuclei, 10% are helium nuclei of alpha particles, and 1% are the nuclei of heavier elements. Solitary electrons constitute much of the remaining 1%.
Def. cosmic rays that originate from astrophysical sources are called primary cosmic rays.
Def. cosmic rays that are created when primary cosmic rays interact with interstellar matter are called secondary cosmic rays.
Def. low energy cosmic rays associated with solar flares are called solar cosmic rays.
Cosmic rays are not charge balanced; that is, positive ions heavily outnumber electrons. The positive ions are
- free protons,
- alpha particles (helium nuclei),
- lithium nuclei,
- beryllium nuclei, and
- boron nuclei.
Def. a nuclear reaction in which a nucleus fragments into many nucleons is called spallation.
Cosmic rays cause spallation when a ray particle (e.g. a proton) impacts with matter, including other cosmic rays. The result of the collision is the expulsion of large numbers of nucleons (protons and neutrons) from the object hit.
Carbon and oxygen nuclei collide with interstellar matter to form lithium, beryllium and boron in a process termed cosmic ray spallation. Spallation is also responsible for the abundances of scandium, titanium, vanadium, and manganese ions in cosmic rays produced by collisions of iron and nickel nuclei with interstellar matter.
"Good agreement is seen between the 14C [...] and 10Be [...] records, which confirms they are indeed measuring changes of the GCR flux, since their respective transport processes from the atmosphere to archive are completely different. After its formation, 14C is rapidly oxidised to 14CO2 and then enters the carbon cycle and may reach a tree-ring archive. On the other hand, 10Be attaches to aerosols and eventually settles as rain or snow, where it may become embedded in a stable ice-sheet archive. The correlation between high GCR flux and cold North Atlantic temperatures embraces the Little Ice Age, which is seen not as an isolated phenomenon but rather as the most recent of around ten such events during the Holocene. This suggests that the Sun may spend a substantial fraction of time in a magnetically-quiet state."[9]
Sources
[edit | edit source]Z → | 0 | 1 | 2 | ||||||||||||
---|---|---|---|---|---|---|---|---|---|---|---|---|---|---|---|
n ↓ | n | H | He | 3 | 4 | ||||||||||
0 | 1H | Li | Be | 5 | 6 | ||||||||||
1 | 1n | 2H | 3He | 4Li | 5Be | B | C | 7 | |||||||
2 | 2n | 3H | 4He | 5Li | 6Be | 7B | 8C | N | 8 | ||||||
3 | 4H | 5He | 6Li | 7Be | 8B | 9C | 10N | O | 9 | ||||||
4 | 4n | 5H | 6He | 7Li | 8Be | 9B | 10C | 11N | 12O | F | 10 | ||||
5 | 6H | 7He | 8Li | 9Be | 10B | 11C | 12N | 13O | 14F | Ne | 11 | ||||
6 | 7H | 8He | 9Li | 10Be | 11B | 12C | 13N | 14O | 15F | 16Ne | Na | 12 | |||
7 | 9He | 10Li | 11Be | 12B | 13C | 14N | 15O | 16F | 17Ne | 18Na | Mg | 13 | |||
8 | 10He | 11Li | 12Be | 13B | 14C | 15N | 16O | 17F | 18Ne | 19Na | 20Mg | Al | 14 | ||
9 | 12Li | 13Be | 14B | 15C | 16N | 17O | 18F | 19Ne | 20Na | 21Mg | 22Al | Si | |||
10 | 14Be | 15B | 16C | 17N | 18O | 19F | 20Ne | 21Na | 22Mg | 23Al | 24Si | ||||
11 | 16B | 17C | 18N | 19O | 20F | 21Ne | 22Na | 23Mg | 24Al | 25Si | |||||
12 | 18C | 19N | 20O | 21F | 22Ne | 23Na | 24Mg | 25Al | 26Si | ||||||
13 | 20N | 21O | 22F | 23Ne | 24Na | 25Mg | 26Al
|
27Si | |||||||
14 | 22O | 23F | 24Ne | 25Na | 26Mg | 27Al | 28Si |
Neutrons are produced when alpha particles impinge upon any of several low atomic weight isotopes including isotopes of lithium, beryllium, carbon and oxygen.
Gamma radiation with an energy exceeding the neutron binding energy of a nucleus can eject a neutron. Two examples and their decay products:
- 9Be + >1.7 Mev photon → 1 neutron + 2 4He
Traditional particle accelerators with hydrogen (H), deuterium (D), or tritium (T) ion sources may be used to produce neutrons using targets of deuterium, tritium, lithium, beryllium, and other low-Z materials. Typically these accelerators operate with voltages in the > 1 MeV range.
Neutrons (so-called photoneutrons) are produced when photons above the nuclear binding energy of a substance are incident on that substance, causing it to undergo giant dipole resonance after which it either emits a neutron (photodisintegration) or undergoes fission (photofission). The number of neutrons released by each fission event is dependent on the substance. Typically photons begin to produce neutrons on interaction with normal matter at energies of about 7 to 40 MeV, which means that megavoltage photon radiotherapy facilities may produce neutron radiation as well, and require special shielding for it. In addition, electrons of energy over about 50 MeV may induce giant dipole resonance in nuclides by a mechanism which is the inverse of internal conversion, and thus produce neutrons by a mechanism similar to that of photoneutrons.[10]
A spallation source is a high-flux source in which protons that have been accelerated to high energies hit a target material, prompting the emission of neutrons.
Nuclear fusion, the combining of the heavy isotopes of hydrogen, also has the potential to produce large quantities of neutrons.
Neutron emission is a type of radioactive decay of atoms containing excess neutrons, in which a neutron is simply ejected from the nucleus. Two examples of isotopes which emit neutrons are beryllium-13 (mean life 2.7x10-21 sec) and helium-5 (7x10-22 sec).
Neutron emission usually happens from nuclei that are in an excited state, such as the excited O-17* produced from the beta decay of N-17. The neutron emission process itself is controlled by the nuclear force and therefore is extremely fast, sometimes referred to as "nearly instantaneous." The ejection of the neutron may be as a product of the movement of many nucleons, but it is ultimately mediated by the repulsive action of the nuclear force that exists at extremely short-range distances between nucleons. The life time of an ejected neutron inside the nucleus before it is emitted is usually comparable to the flight time of a typical neutron before it leaves the small nuclear "potential well," or about 10-23 seconds.[11] A synonym for such neutron emission is "prompt neutron" production, of the type that is best known to occur simultaneously with induced nuclear fission. Many heavy isotopes, most notably californium-252, also emit prompt neutrons among the products of a similar spontaneous radioactive decay process, spontaneous fission.
Most neutron emission outside prompt neutron production associated with fission (either induced or spontaneous), is from neutron-heavy isotopes produced as fission products. These neutrons are sometimes emitted with a delay, giving them the term delayed neutrons, but the actual delay in their production is a delay waiting for the beta decay of fission products to produce the excited-state nuclear precursors that immediately undergo prompt neutron emission. Thus, the delay in neutron emission is not from the neutron-production process, but rather its precursor beta decay which is controlled by the weak force, and thus requires a far longer time. The beta decay half lives for the precursors to delayed neutron-emitter radioisotopes, are typically fractions of a second to tens of seconds.
Alloys
[edit | edit source]Beryllium copper (BeCu), also known as copper beryllium (CuBe), beryllium bronze and spring copper, is a copper alloy with 0.5–3% beryllium,[12] but can contain other elements as well. Beryllium can be alloyed with nickel and aluminum.[13]
Materials
[edit | edit source]Beryllium occurs in a hexgonal close-packed (hcp) crystal structure at room temperature (α-Be).
As indicated in the phase diagram on the left beryllium occurs as (β-Be) which is bcc at higher temperatures up to melting.
Native beryllium is not known to occur on the surface of the Earth, but may eventually be found among beryllium-bearing minerals in small amounts.
Minerals
[edit | edit source]Behoites
[edit | edit source]The natural pure beryllium hydroxide is rare (in form of the mineral behoite, orthorhombic) or very rare (clinobehoite, monoclinic).[14][15]
Meteorites
[edit | edit source]
Moldavite is an olive-green or dull greenish vitreous substance possibly formed by a meteorite impact. It is one kind of tektite.
Because of their difficult fusibility, extremely low water content, and its chemical composition, the current overwhelming consensus among Earth scientists is that moldavites were formed 15 million years ago during the impact of a giant meteorite in present-day Nördlinger Ries. Splatters of material that was melted by the impact cooled while they were actually airborne and most fell in central Bohemia—traversed by [the] Vltava river. Currently, moldavites have been found in [an] area that includes southern Bohemia, western Moravia, the Cheb Basin (northwest Bohemia), Lusatia (Germany), and Waldviertel (Austria).[16] Isotope analysis of samples of moldavites have shown a beryllium-10 isotope composition similar to the composition of Australasian tektites (Australites)and Ivory Coast tektites (Ivorites). Their similarity in beryllium-10 isotope composition indicates that moldavites, Australites, and Ivorites consist of near surface and loosely consolidated terrestrial sediments melted by hypervelocity impacts.[17]
Petrochemistry
[edit | edit source]Many rocky objects are composed of oxide minerals. Oxygen has three known stable isotopes: 16O, 17O, and 18O.
"The stable isotopic compositions of low-mass (light) elements such as oxygen, hydrogen, [helium, lithium, beryllium, boron,] carbon, nitrogen, [fluorine, neon, sodium, magnesium, aluminum, silicon, phosphorus,] and sulfur are normally reported as "delta" ([δ]) values in parts per thousand (denoted as ‰ [per mille]) enrichments or depletions relative to a standard of known composition."[18]
For 18O to 16O:
The "ratio [by convention is] of the heavy to light isotope in the sample or standard."[18]
"Various isotope standards are used for reporting isotopic compositions; the compositions of each of the standards have been defined as 0‰. Stable oxygen and hydrogen isotopic ratios are normally reported relative to the SMOW standard ("Standard Mean Ocean Water" (Craig, 1961b)) or the virtually equivalent VSMOW (Vienna-SMOW) standard. Carbon stable isotope ratios are reported relative to the PDB (for Pee Dee Belemnite) or the equivalent VPDB (Vienna PDB) standard. The oxygen stable isotope ratios of carbonates are commonly reported relative to PDB or VPDB, also. Sulfur and nitrogen isotopes are reported relative to CDT (for Cañon Diablo Troilite) and AIR (for atmospheric air), respectively."[18]
"Ordinary water consists of slightly different kinds of so-called isotopic water molecules of equal chemical properties but different masses: a light one (H2O16), which occurs most frequently by far in natural waters, and quite a few heavier ones, of which the H2O18 and the [deuterium, D) HDO16 components occur in concentrations of approximately 2000 and 320 ppm (parts per million) water molecules, respectively. Due to slightly different vapour pressures and rates of reaction, the concentrations of the isotopic components change somewhat during phase-shifts in the natural water cycle".[19]
Ice "cores contain a wealth of information on past climates in the form of a great number of parameters, of which some are listed below:"[19]
- Concentrations of the oxygen-18 and deuterium components of water in the ice give information about the cloud temperature and precipitation at the time of deposition,
- the content of air in bubbles reveals the altitude of the then ice surface,
- the concentrations of carbon dioxide and methane in the air bubbles tell about the greenhouse effect in past atmospheres,
- the chemical composition of the ice itself gives information about other aspects of the chemistry in past atmospheres,
- dust and calcium concentration tell about the violence and frequency of the storms that carried dust from ice free areas to the inland ice, and
- the acidity of the ice indicates the fall-out of volcanic acids and thereby past volcanic activity.
Stars
[edit | edit source]"The atmospheres of the two program stars (HR 6158 = 28 Her = HD 149212; HR 8915 = 69 Peg = HD 220933) contain an inordinate amount of beryllium (Be); in fact, the Be abundances in these stars are among the highest known. ... lithium (Li) is detected in neither HR 6158 nor HR 8915."[20]
Resources
[edit | edit source]See also
[edit | edit source]References
[edit | edit source]- ↑ Thomas K. Gaisser (1990). Cosmic Rays and Particle Physics. Cambridge University Press. pp. 279. http://books.google.com/books?hl=en&lr=&id=qJ7Z6oIMqeUC&oi=fnd&pg=PR15&ots=IxjwLxBwXu&sig=voHKIYstBlBYla4jcbur_b-Zwxs. Retrieved 2014-01-11.
- ↑ Willard Lincoln Roberts; George Robert Rapp Jr.; Julius Weber (1974). Encyclopedia of Minerals. New York, New York, USA: Van Nostrand Reinhold Company. pp. 693.
- ↑ P Sielicki; H Janik; A Guzman (2012). "Grain type and size of particulate matter from diesel vehicle exhausts analysed by transmission electron microscopy". Environmental Technology 33 (15). doi:10.1080/09593330.2011.646315. http://www.tandfonline.com/doi/abs/10.1080/09593330.2011.646315. Retrieved 2013-08-29.
- ↑ Lalovic, M.; Werle, H. (1970). "The energy distribution of antimonyberyllium photoneutrons". Journal of Nuclear Energy 24 (3): 123–132. doi:10.1016/0022-3107(70)90058-4.
- ↑ Ahmed, S. N. (2007). Physics and Engineering of Radiation Detection. p. 51. ISBN 978-0-12-045581-2. Bibcode: 2007perd.book.....A. https://books.google.com/books?id=3KdmdcGbBywC&pg=PA51.
- ↑ Handbook on Photonuclear Data for Applications: Cross-sections and Spectra. IAEA. http://www-pub.iaea.org/books/iaeabooks/6043/Handbook-on-Photonuclear-Data-for-Applications-Cross-sections-and-Spectra.
- ↑ Pol098 (22 July 2005). cosmic ray. San Francisco, California: Wikimedia Foundation, Inc. https://en.wiktionary.org/wiki/cosmic_ray. Retrieved 21 April 2022.
- ↑ S. Y. Lee (2004). Accelerator physics, Second Edition. Singapore: World Scientific Publishing Co. Pte. Ltd.. pp. 575. ISBN 981-256-182-X. http://books.google.com/books?id=VTc8Sdld5S8C&lr=&source=gbs_navlinks_s. Retrieved 2011-12-17.
- ↑ Jasper Kirkby (November 01, 2007). "Cosmic rays and climate". Surveys in Geophysics 28 (5-6): 333-75. doi:10.1007/s10712-008-9030-6. http://arxiv.org/pdf/0804.1938.pdf. Retrieved 2014-09-19.
- ↑ [1]
- ↑ Neutron emission lifetime and why. http://newenergytimes.com/v2/library/2000/2000Li-Sub-BarrierFusion.pdf. Retrieved 2012-09-17.
- ↑ http://www.matthey.ch/index.php?id=45&L=1 Copper beryllium and nickel beryllium datasheets.
- ↑ "Beralcast – Beryllium Aluminum Alloys". IBC Advanced Alloys. Retrieved 2015-07-22.
{{cite web}}
:|archive-date=
requires|archive-url=
(help) - ↑ Mindat, http://www.mindat.org/min-603.html
- ↑ Mindat, http://www.mindat.org/min-1066.html
- ↑ Trnka, M.; Houzar, S. (2002). "Moldavites: a review PDF". Bulletin of the Czech Geological Survey 77 (4): 283–302. http://www.geology.cz/bulletin/contents/2002/vol77no4/04trnkafinal.pdf.
- ↑ Serefiddin, F.; Herzog, G. F.; Koeberl, C. (2007). "Beryllium-10 concentrations of tektites from the Ivory Coast and from Central Europe: Evidence for near-surface residence of precursor materials". Geochimica et Cosmochimica Acta 71 (6): 1574–82. http://www.univie.ac.at/geochemistry/koeberl/publikation_list/296-Be-10-Ivory-Coast-tektites-and-moldavites-GCA2007.pdf.
- ↑ 18.0 18.1 18.2 Carol Kendall (January 2004). Resources on Isotopes. Menlo Park, California USA: United States Geological Survey. http://wwwrcamnl.wr.usgs.gov/isoig/res/funda.html. Retrieved 2014-10-05.
- ↑ 19.0 19.1 Willi Dansgaard (2005). The Department of Geophysics of The Niels Bohr Institute for Astronomy Physics and Geophysics at The University of Copenhagen Denmark. ed. Frozen Annals Greenland Ice Cap Research. Copenhagen, Denmark: Niels Bohr Institute. pp. 123. ISBN 87-990078-0-0. http://www.iceandclimate.nbi.ku.dk/publications/FrozenAnnals.pdf/. Retrieved 2014-10-05.
- ↑ Alex Stephens; Constantine P. Deliyannis (April 1999). "Lithium in the Super–Beryllium‐rich Hg‐Mn Stars HR 6158 and HR 8915". Publications of the Astronomical Society of the Pacific 111 (758): 482-8. doi:10.1086/316344. http://www.jstor.org/stable/10.1086/316344. Retrieved 2013-07-14.