1. Introduction
Improved education of the public about the risk factors of subarachnoid hemorrhage (SAH) along with the increasingly more sophisticated medical culture of the digital age resulted in a remarkable decrease in SAH incidence from ~10 per 100,000 person-years in 1980 to ~6 per 100,000 in 2010 [1]. Yet, this still translates to a current global prevalence of ~8 million people suffering from SAH [2]. Importantly, severe cases of SAH with IV and V grades on the World Federation of Neurosurgical Societies (WFNS) scale, corresponding with 3–12 grades of the Glasgow Coma Scale (GCS), still have a high, more than 50%, mortality rate even in hospital environments: an outcome virtually unchanged since the 1960s [3, 4]. Among the survivors of SAH, the morbidity rate is substantial with at least 40% of these patients remaining disabled and dependent for more than a year whether in rehabilitation centers or at home [5].
These facts highlight the progress in SAH treatment in the last decades, as a consequence of the highly improved diagnostic repertoire, available drugs, and neurosurgical interventions in this period. But the other message of these facts is that SAH is still a devastating condition for the millions affected by its severe forms, either traumatic or nontraumatic, aneurysmal or nonaneurysmal. Clearly, basic science research for SAH must be the most creatively expanded, just as further improvements on the available diagnostic, pharmacological, and neurosurgical approaches must also be vigorously pursued. At the same time, the unsolved problem of medically meaningful therapy for WFNS IV-V grade SAH calls for the development and testing of new, even radically new, therapeutic methods and technologies.
The objective of the present paper is to evaluate the clinical viability of such a new therapeutic method and technology, the long-term use of a drug delivery, neurotoxin drainage, and EEG recording system partly implantable in the neurocranium and partly in the subarachnoid space. It is the NPD, which is extensively described and tested preclinically for neocortical seizure prevention [6–11] and readily adaptable for SAH.
2. Methods
The clinical viability of anti-SAH NPD was evaluated by reexamining the related neuroscientific and clinical publications, considering the medical inventions these publications inspired, taking a deeper look into the theoretical aspects of this technology and placing each into the context of relevant studies by other investigators.
The idea of an NPD-like, EEG-equipped, intracerebral drug-delivery device was originally described in a book chapter and a related US Patent in 2000 and 2002, respectively [6, 7]. Both argued that since the neurons of the brain work as hybrid, chemically controlled electrical computers, their dysfunctions, including those behind otherwise intractable neurological and psychiatric disorders, must ideally be corrected with brain implants that are able to deliver therapeutic chemical compounds into the very sites of dysfunction. The superiority of brain implants over bedside systems was explained by pointing out that implants have the ability to provide long-term care: an absolute necessity for treating chronic brain disorders. This idea was later translated into increasingly better-functioning brain implants tested in freely behaving non-human primates to generate data relevant to humans. These studies clarified that for brain disorders with predominantly cerebral cortical dysfunctions localized subarachnoid drug delivery is more effective and safer than drug delivery though cannulas or catheters led into the cerebral cortical tissue [8, 9]. Furthermore, the studies clarified that alternating drug deliveries with subarachnoid cerebrospinal fluid (CSF) drainage is critical for both preventing clogging in the drug-delivery system and optimizing the effects of drug delivery with the removal of harmful endogenous molecules from the interstitial space [8, 9]. It was also proved that these subarachnoid drug delivery–CSF drainage devices could function safely, leaving the health, behavior, motor, and memory functions of the implanted monkeys intact in year-long studies [10]. Thus, the potential of this device to provide long-term care has been proved.
The necessity of monitoring the safety and efficacy of the device with an integrated EEG system—a system adaptable to seizure-diagnostic headsets—was shown as well [8]. These preclinical studies focused on the antiepileptic efficacy of the device with one study in a clinical neurosurgical setting proving the efficacy of subarachnoid (“transmeningeal”) drug delivery to inhibit focal neocortical seizures in human epileptic patients [11].
When the indicated results were placed in the context of relevant studies from other investigators by using the PubMed database, the limited work on drug-delivery devices in the field of SAH research was striking. While the keywords “subarachnoid hemorrhage” yielded 36,922 related publications from 1988 through 2023 and the keywords “drug delivery devices” yielded 52,374 ones in the same period, combining them resulted in only 23 papers. Of these 23 papers, 16 were found to be irrelevant ones dealing with in vitro studies, non-pharmacological SAH treatments or drug deliveries for other brain disorders.
Nevertheless, seven papers did report important advances in the use of intracerebral drug-delivery systems for SAH treatment. They described both animal and human studies using cisternal/intrathecal diltiazem, papaverine or cromakalim delivery [12, 13], subarachnoid delivery of sustained-release nimodipine microparticles [14, 15], and intracerebral delivery of vascular endothelial growth factor (VEGF) and paclitaxel via drug-releasing stents [16], all against SAH-induced vasospasm. Most remarkably, as a culmination of their earlier research, Carlson and his team reported a few months ago, in March 2023, that for SAH treatment “Combined CSF drainage, irrigation, multimodality monitoring, and automated local drug delivery are feasible using twist-drill hole device”. They emphasized that “Further investigation of irrigation settings and treatment approaches in high-risk cases is warranted” [17, 18].
Since the NPD technology specifically aims to treat high-grade SAH patients selected for decompressive craniotomy or craniectomy, the above PubMed search was extended to this topic. Decompressive craniotomy and decompressive craniectomy yielded 3,730 and 3,394 relevant publications, respectively. A closer look into them showed that though the use of these procedures for traumatic and nontraumatic intracranial hemorrhages has been controversial [19], craniectomy, including bilateral craniectomy [20], can achieve favorable, often life-saving, outcomes in a significant number of patients with severe SAH [21].
3. Results
All of the above SAH studies by other teams testing drug-delivery devices and direct intracranial drug applications [12–18] reported beneficial, potentially therapeutic effects. For example, clinical studies with SAH patients showed that application of vasodilators diltiazem and papaverine through cisternal drainage tubes effectively reduced post-SAH vasospasm [12] and intracerebroventricular applications of sustained release nimodipine microparticles [15] “was associated with reduced delayed cerebral ischemia (DCI)” [15].
Though our epilepsy-centered NPD work was not extended to active stroke research, the potential of the device in treating cerebrovascular disorders was examined and proposed [22]. Specifically, we wrote that “for those with hemorrhagic strokes, a viable therapeutic option would be to replace their cranial bone over the area of stroke with bioengineered, multifunctional bone. Such a device-carrying bone can deliver necessary neurotrophic factors and edema reducers along with drugs and vectors…to promote neuroregeneration, neuroplasticity and neurogenesis, especially for stroke patients with decompressive hemicraniectomy” [22].
The design and functions of such devices were patented [23], and in a recent paper the distant future of the implant was also discussed [24].
Belonging to the cited efforts by others [12–18] to empower SAH therapy with intracranial drug delivery and CSF drainage, the NPD for SAH and SAH-induced vasospasm does offer three distinguishing features. These are:
Craniectomy-closing device implantation. Although this feature limits the use of NPD for craniectomy-requiring, WFNS IV-V grade SAH cases, it would give hope to thousands of Americans and tens of thousands worldwide suffering from these life-threatening conditions.
Synergy of blood-brain barrier (BBB)-bypassing subarachnoid polypharmacy and CSF cleaning. While this aims to use localized, intracranial polypharmacy to simultaneously reduce the risk of rebleeding (e.g., with TNFα inhibitors or better agents), prevent vasospasm (e.g., with endothelin-1 receptor antagonists, eNOS mRNAs, or better agents), and provide neuroprotection in DCI (e.g., with erythropoietin or better agents), critically important synergy is also produced by the alternation of these drug applications with CSF drainage cleaning the subarachnoid environment and its cortical extracellular channels from excess of proinflammatory cytokines [25], excitotoxic glutamate [26], and other harmful endogenous molecules, as well as cellular debris, including vasospasm-inducing breakdown products of blood [27].
Long-term treatment regularly adjusted by EEG and brain chemistry data. Since the NPD has EEG recording capacity and its drained fluids can be periodically removed and processed for clinical laboratory assays, the information derived from these two sets of data can help the treating physician to continuously adjust the parameters of drug delivery and CSF drainage. In turn, this should lead to the right treatment in each phase of SAH.
The design of anti-SAH NPDs is shown in Figure 1.
Figure 1
(a) Spatial relationship between the NPD control unit and its connected subarachnoid strip. Note that the control unit—which integrates a battery, a delivery minipump with drug reservoir, a drainage minipump with CSF reservoir, a four-channel EEG system, a wireless communication module, and the necessary processor and controller electronics—closes the craniectomy without interfering its decompressing effect. Also note the subcutaneous ports to monthly/quarterly refill and empty the drug and drainage reservoirs, respectively. (b) Functions of the subarachnoid strip, as indicated.
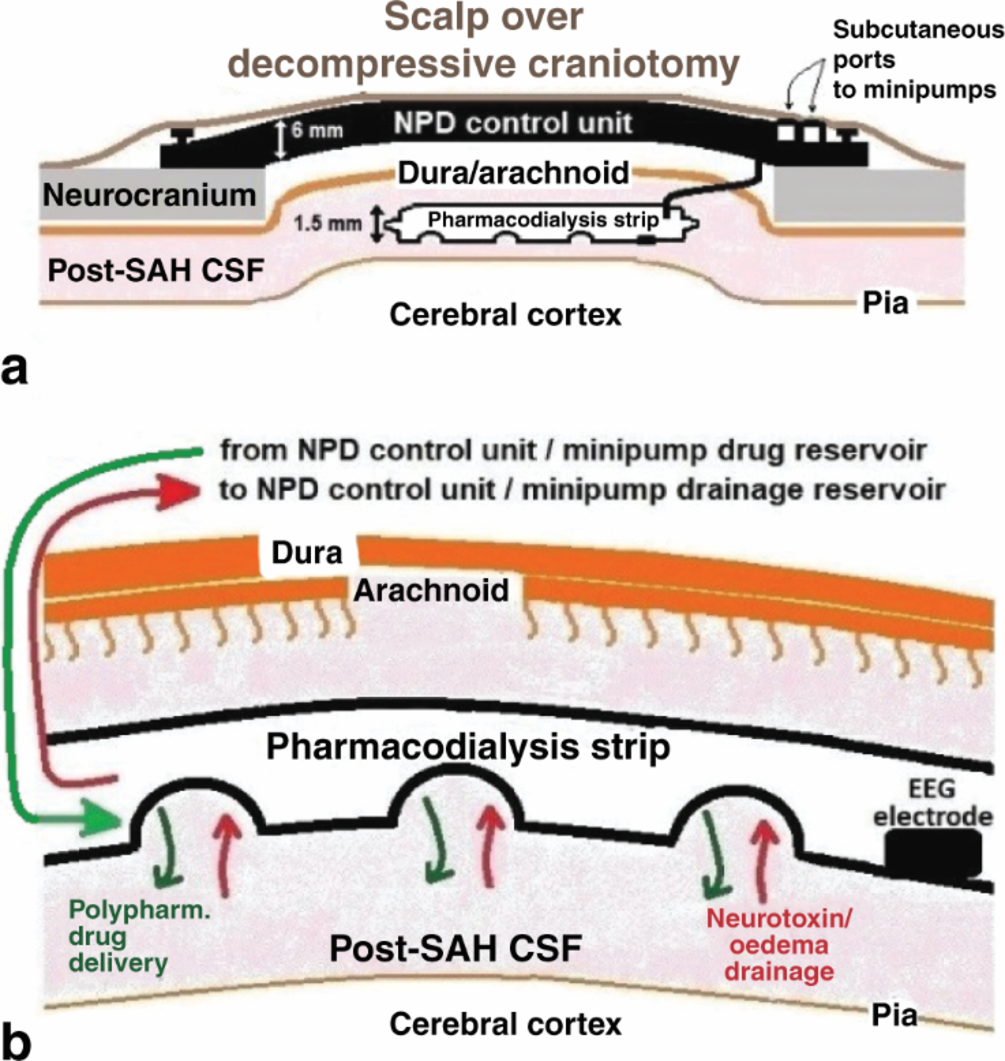
Whether the implantation and use of this—constantly improved—device was safe enough for proposing its introduction into neurological care was examined in a decade-long work by us [8–10]. It was found that the non-human primates implanted and treated with this device kept their physical and mental health throughout these year-long studies, as demonstrated in Figure 2.
Figure 2
Photograph of bonnet macaques implanted with the antiepileptic NPD version during their 8th month (Monkey 1) and 12th month (Monkey 2) of subarachnoid delivery of 1.0 mM muscimol twice a day for the prevention of experimental focal seizures. Drug deliveries were alternating with CSF drainage. The animals’ behavior was indistinguishable from normal, just as their transmitted EEG. The ~ 90 g monkey brain made it necessary to secure the control unit extracranially under a cap. The ~1,400 g human brain makes this unnecessary. (Adapted from Ludvig [10], page 78, Fig. 8, Copyright 2012, with permission from Bentham Science Publishers).
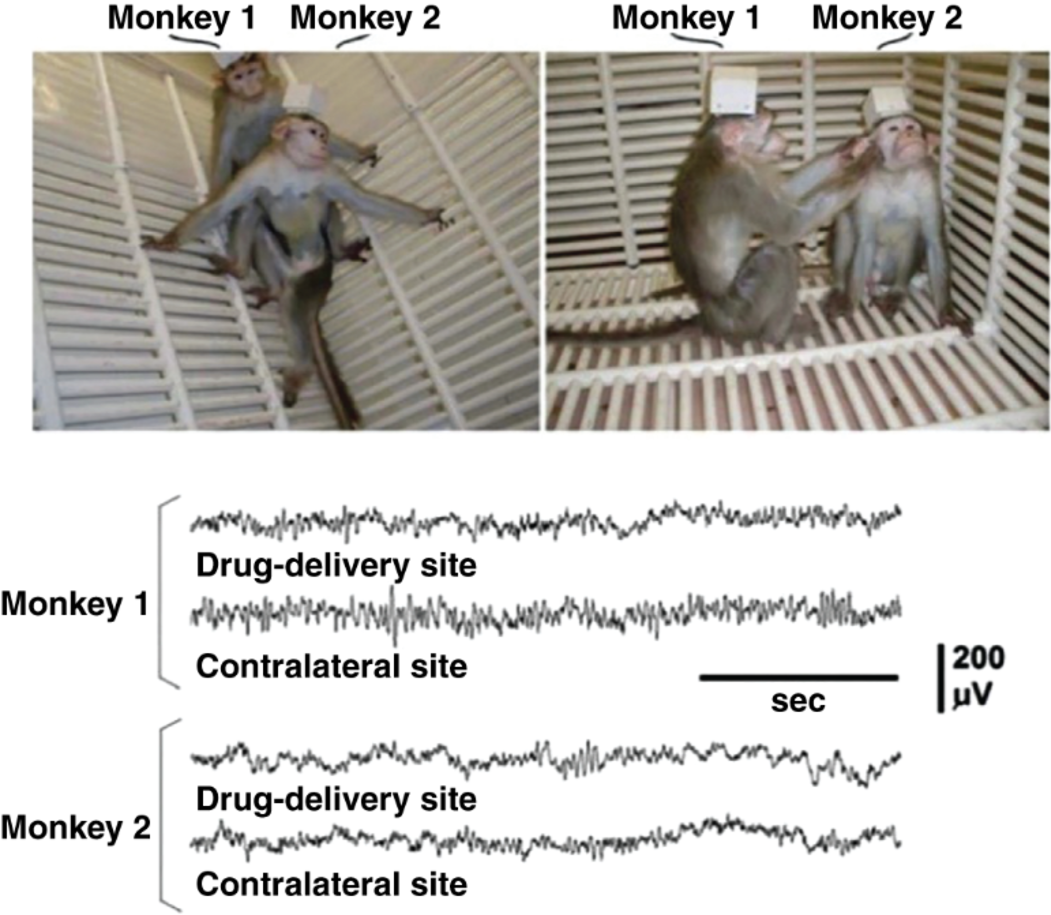
4. Discussion
SAH patients reaching the hospital alive with WFNS grade IV-V conditions face the dire complications of not just the original bleeding but also of rebleeding, cerebral vasospasm, DCI, and hydrocephalus, a mortal combination in about 50% of them with most of the rest becoming disabled, dependent survivors for many years. To change this situation, conservative medical measures and interventional options must be further improved and new, even radically new, treatments must also be explored. NPD technology is one of these potential new treatments. It is a neurocranial device with subarachnoid connections to perform the multiple functions of subarachnoid drug delivery, edema drainage, and neurotoxin dialysis with EEG feedback. It has advantages and disadvantages, as detailed in Table 1.
Whether these advantages of NPD use will inspire the work needed to introduce this medical invention into SAH therapy or, instead, the disclosed disadvantages will be viewed as too discouraging to accomplish that work; it is a choice for the future. For this choice, the following practical information can be used: the antiepileptic version of the NPD reached the FDA in 2019 as a “Subdural Pharmacotherapy Device for the treatment of currently intractable focal epilepsies” and was assigned to the Office of Combination Products (OCP) of the Center for Drug Evaluation and Research (CDER) on the grounds that the Primary Mode of Action (PMOA) is pharmacological. Due to a lack of funds, FDA regulatory approvals for this drug-delivery device were not pursued further at the time. However, this process can be readily restarted for a “Neurocranial Pharmacodialysis Device for the treatment of severe Subarachnoid Hemorrhage”. In fact, since high-grade SAH affects less than 8,000 individuals in the United States per year, the anti-SAH NPD would qualify as an Orphan Product eligible for R&D funds from the Office of Orphan Product Development (OOPD) of the FDA. Should OOPD grants and private donations be adequate for the remaining R&D work and exploratory clinical trials for the anti-SAH NPD, the price of the basic device for unilateral craniectomy could be kept as low as ~$24,000, a sum providing no financial gain for this author while including the presently unavoidable profit of 20% of this price for the lead manufacturer.
Table 1
Advantages and disadvantages of possible NPD use for SAH
Advantages |
|
Disadvantages |
|
5. Conclusions
It is reasonable to suggest that complementing conservative therapies with NPD implantation can reduce the current substantial mortality and morbidity among hospitalized survivors of SAH and post-SAH vasospasm. While the safe and effective use of the antiepileptic version of the device was demonstrated in year-long experiments in nonhuman primates, and the subarachnoid (“transmeningeal”) method of its drug delivery worked in human patients in a clinical neurosurgical setting, an adaptation of the technology to high-grade SAH patients, especially those whose treatment requires decompressive craniectomy, has yet to be accomplished.