Volume 18 Issue 4 Summer 2022www.nasa.govNationalAeronauticsand Space Administration
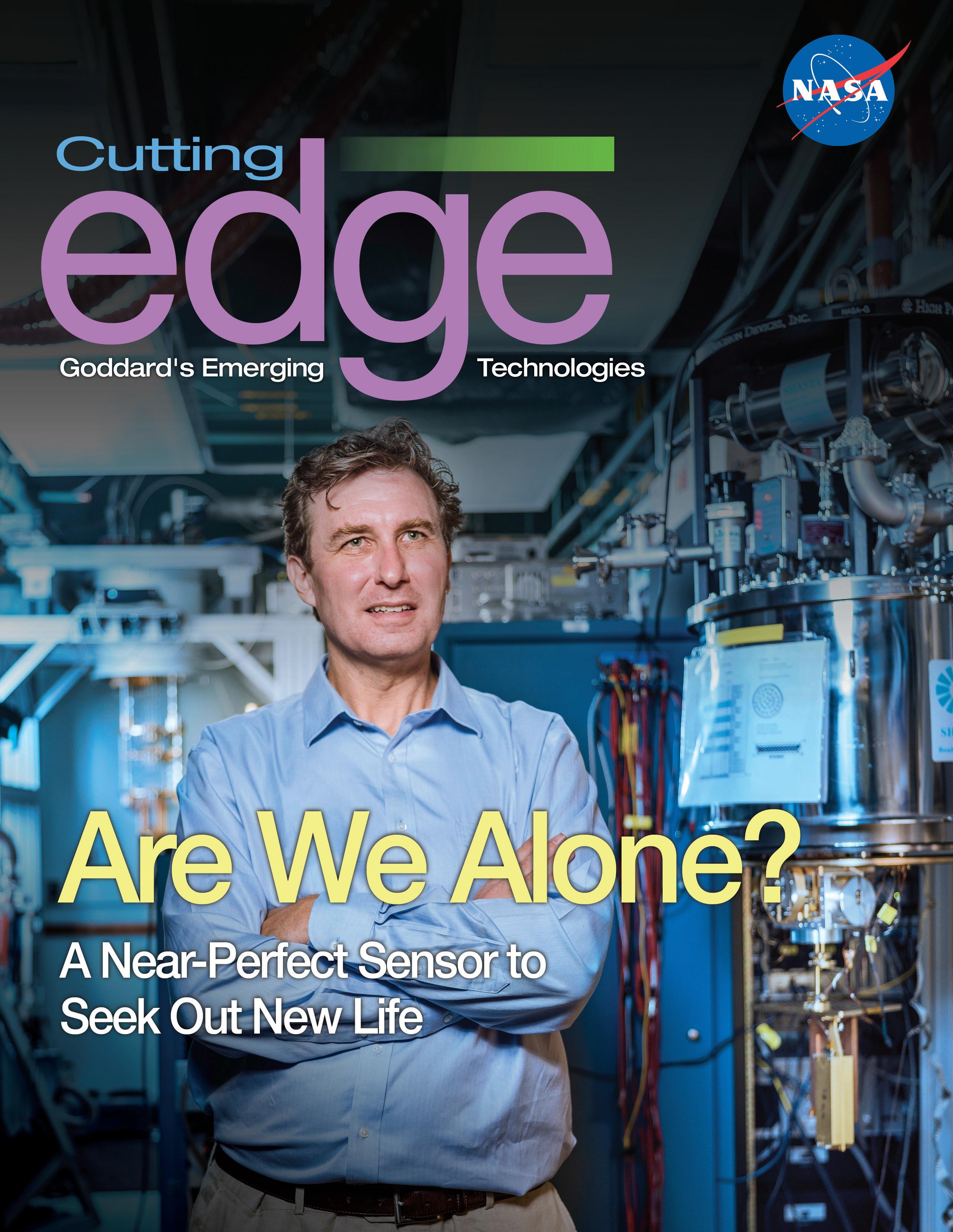
–
(Image Credit: NASA/Chris Gunn) 2 Goddard’s
Goddard innovators utilizing classical quantum effects – the unique properties and behaviors of materials at the atomic level – are developing a
About the Cover Jack Sadleir stands in his lab with his sensor technology suspended in a cryogenic testing setup. His tiny sensor, powered by quantum waveform effects, not only detects incoming photons with unmatched precision, but can tell what color of light each photon is. Quantum technologies taking advantage of the seemingly impossible physics of matter at the atomic level and smaller could change the way NASA communicates, collects science data, explores and even lands humans safely on other worlds. Innovations in Quantum Technology World as a Model Exoplanet Gravity By the Atoms Geodesy Innovation Born at Goddard Communications Developing Capabilities for the Future Talent for Quantum Investigations Quantum Dots Enable Spacecraft-as-Sensor Concept High-Powered Laser to Find Water on the Moon in this issue: Mastering the peculiar behaviors of matter and light at the smallest measurable levels changes the way scientists observe and explore the universe at the grandest scales. Scientists have refined understanding of quan tum physics, the properties of matter and energy at the atomic and sub-atomic scale, for more than 100 years. These investigations are beginning to yield technological solutions, said Renee Reynolds, Goddard’s assistant chief technologist for Instru ment mechanics, entanglement and superposition promise to enable transformational science applications in the areas of quantum sensing, quantum communications and networking, and atom interferometry,” she said. “Here at God dard, we aim to build upon external partnerships and internal expertise to develop next generation quantum technologies and infrastructures.”
“PrinciplesSystems.ofquantum
technologiesemerginggoddard’sedgecutting• PAGE 2 Volume 18 • Issue 4 • Summer 2022 www.nasa.gov/gsfctechnology
Goddard’s Innovations in Quantum Technology
13
Summer2022
Manipulating entangled photons promises instan taneous communication unbound by the speed of light over vast distances. Even low-volume data –bits or kilobits – offers promise for unbreakable data encryption keys
–
our
> Satellite
4 Our
15 Tiny,
powerful new generation of detectors. These applications promise more powerful space and ground telescopes, more compact sensors to measure chemicals and composition of other planets, and instruments to directly measure gravity itself and how it changes over time and space.
7 Measuring
On another level – often referred to as quantum 2.0 – the capability of a particle or photon to exist in more than one state or position simultaneously, or superposition, opens new possibilities for commu nications, encryption, and computers exponentially more powerful than currently possible. Superposi tioned particles exhibit a trait called entanglement meaning any changes to the state of one of a pair instantaneously affects its entangled twin, regard less the distance between them.
10 Quantum
> Growing
–

As quantum physics moves from theory to practice, Goddard is well situated to engage in developing quantum technology and applications.
“This is the place to come to work if you want to be involved in quantum technologies,” Goddard Chief Technologist Peter Hughes said, “especially as we take them into space and put them to work in exploring the universe.”
Quanta of Collaboration
v Extremely small materials like the quantum dot crystal illustrated here alter the way basic particles such as photons and electrons function within their boundaries. The very size of these materials limits how atoms, particles, and photons behave within the crystal, sometimes seeming to break the laws of physics. Goddard researchers are putting these and other quantum functions and materials to work in groundbreaking technological applications.
PolandTechnology,ofUniversityPoznanPiatkowski,Sterl/LukaszFloriancredit:Image
Entangled pairs of photons gener ated by an orbiting laser could enable powerful image-correcting optics. This could cancel out the distortion of Earth’s turbulent atmosphere and expand the vision of telescopes on the ground deeper into the cosmos.
Goddard’s global leadership in sensor, communications, and other technolo gies is inextricably linked with partner
Dr. Peter Brereton has been asked to co-lead the Quantum Sensors working group, Reynolds said. “This is a great next step in our future engagement with the broader quantum sensing Membershipcommunity.”inthealliance builds on Goddard’s long tradition of collaborating with universities and other partners, said Joanne Hill, Goddard’s Deputy Director of Engineering and Technology. “Trying to get outside our walls is really important in order to expose graduate students in the sciences and engineering to the work being done here,” Hill said. “Even at NASA, we have to actively recruit, because not everybody is aware of all the players and Innovatorsapplications.”overthe decades established Goddard as a beacon for researchers hungry to test quan tum technology on important science questions in space, Hill said. “At the engineering level, however, there are fewer opportunities out there to work with these technologies. Bringing scientists and technologists closer together and engaging engineers early on is crucial.”
Quantum-powered sensors promise to overcome a natural barrier to resolving the most distant objects in the universe known as the Rayleigh limit. In astrono my, larger mirrors are better at resolving distant, faint light sources up to this limit dictated by the wavelength of the light collected. Quantum sensors could allow future telescopes as powerful as the Hubble Space Telescope to fit into SmallSat packages the size of a com mon countertop appliance.
“Ourinstitutions.recent membership into the Mid-Atlantic Quantum Alliance (MQA) provides a strategic op portunity to build stronger partnerships with our regional quantum ecosystem,” Reynolds said. Facilitated by the University of Maryland, College Park, the alliance serves as a hub for quantum technology research, development, innovation and education organizations. MQA brings together expertise from across academia, industry, govern ment, and research laboratories with the goal of ac celerating innovation across the range of quantum Goddardtechnologies.physicist
Volume 18 • Issue 4 • Summer 2022 technologiesemerginggoddard’sedgecutting• PAGE 3
www.nasa.gov/gsfctechnology
technologiesemerginggoddard’sedgecutting•
Quantum computers may someday soon be capable of calculating faster than physically possible by convention al computers dependent on ones and zeros: the presence or absence of an electrical charge in a single capacitor.
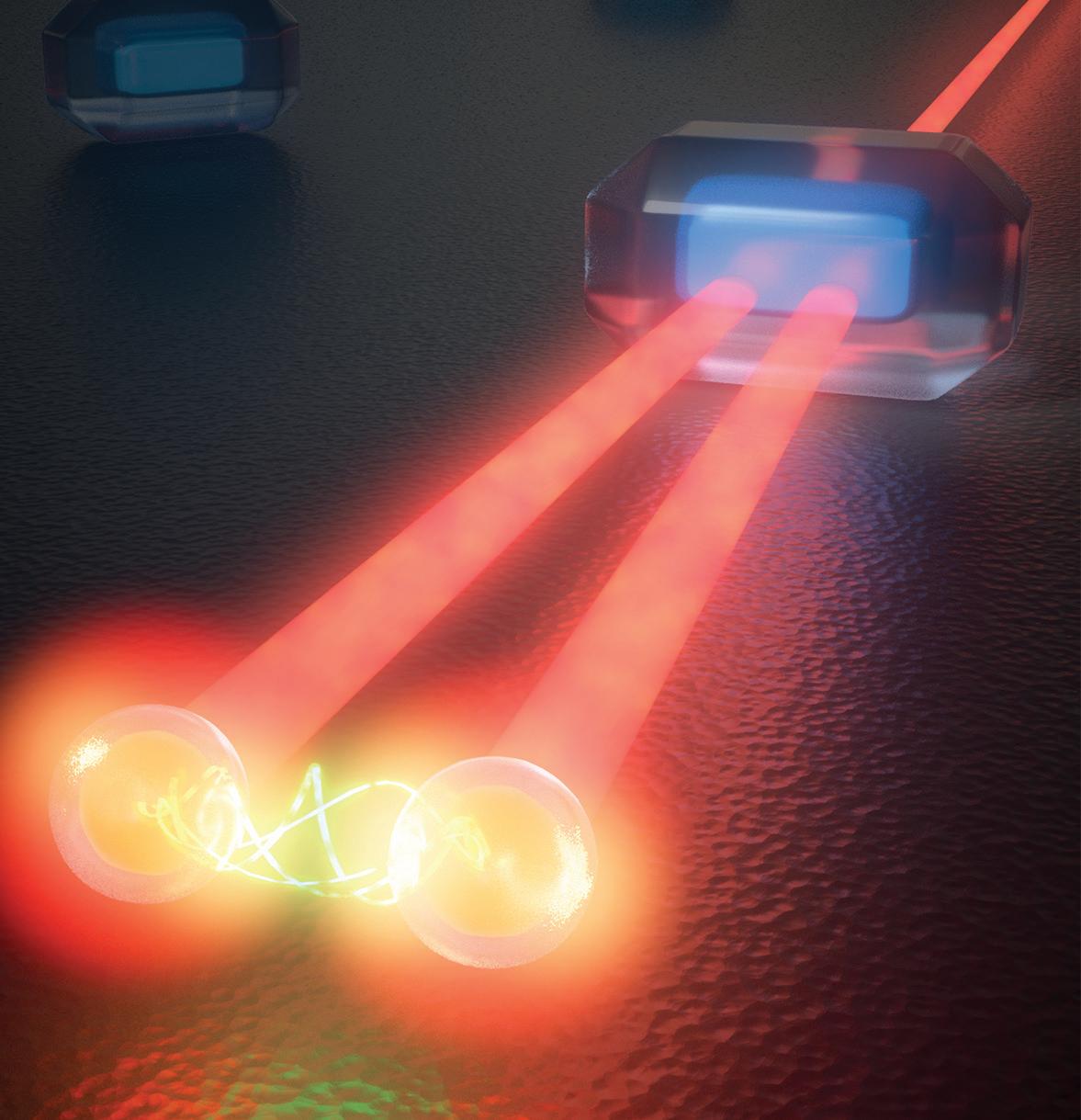
Hunt for Life on Other Planets Requires Patience, Near-Perfect Sensor
“So lower-energy visible photons are red, and as you increase the energy, they go from red to or ange, yellow, green, blue, and violet.”
Astronomers have one ex ample of a world support ing life to help develop and inform the search for life on other planets. Specific col ors of Earth’s light as seen from space reveal an array of chemicals that support life or indicate biological Scientistsprocesses.can also analyze the colors of light from an exoplanet – one orbiting another star – but do ing so presents two hard problems, said Goddard research physicist Jack Sadleir. The host star will be billions of times brighter than the starlight reflect ing off its planets, so direct starlight must be blocked out as much as possible. Even then, the light from the planets will be extreme ly “It’sfaint.an extremely photonstarved observation,” Sadleir said. “You need to collect light efficiently, because for some targets we would point our tele scope and have it stare for weeks. We would just see a slow trickle of photons that would come in, and over time we build up a spectrum, a fingerprint tell ing us the chemistry present on this distant world.
PAGE 4 www.nasa.gov/gsfctechnology
Volume 18 • Issue 4 • Summer 2022
Goddard.Imagecredit:NASA/ChrisGunn
Our World as a Model Exoplanet
Acting like a very precise thermometer, Sadleir’s quantum calorimeter measures how much each photon’s energy heats the sensor.
“In the visible part of the spectrum, that energy cor responds to the photon’s color,” Sadleir explained.
technologiesemerginggoddard’sedgecutting•
If there’s oxygen, water, ozone, or a methane line, together these chemicals could suggest potential signs of life.” Sadleir and his team developed a sensor that not only detects individual and multiple photon hits with unprecedented accuracy but identifies the energy, or color, for each photon detected. When a photon strikes a surface, its energy heats the impact site.
His sensor has demonstrated sensitivity in visible, ultraviolet, and near-infrared wavelengths, and by adjusting the absorber and detector plate or using different materials, Sadleir said detectors can even be manufactured to identify energy from non-elec tromagnetic sources.
“Because we measure deposited energy more genJack Sadleir inspects fiber-optic connections in a cryogenic system used to test his life-finding sensors at
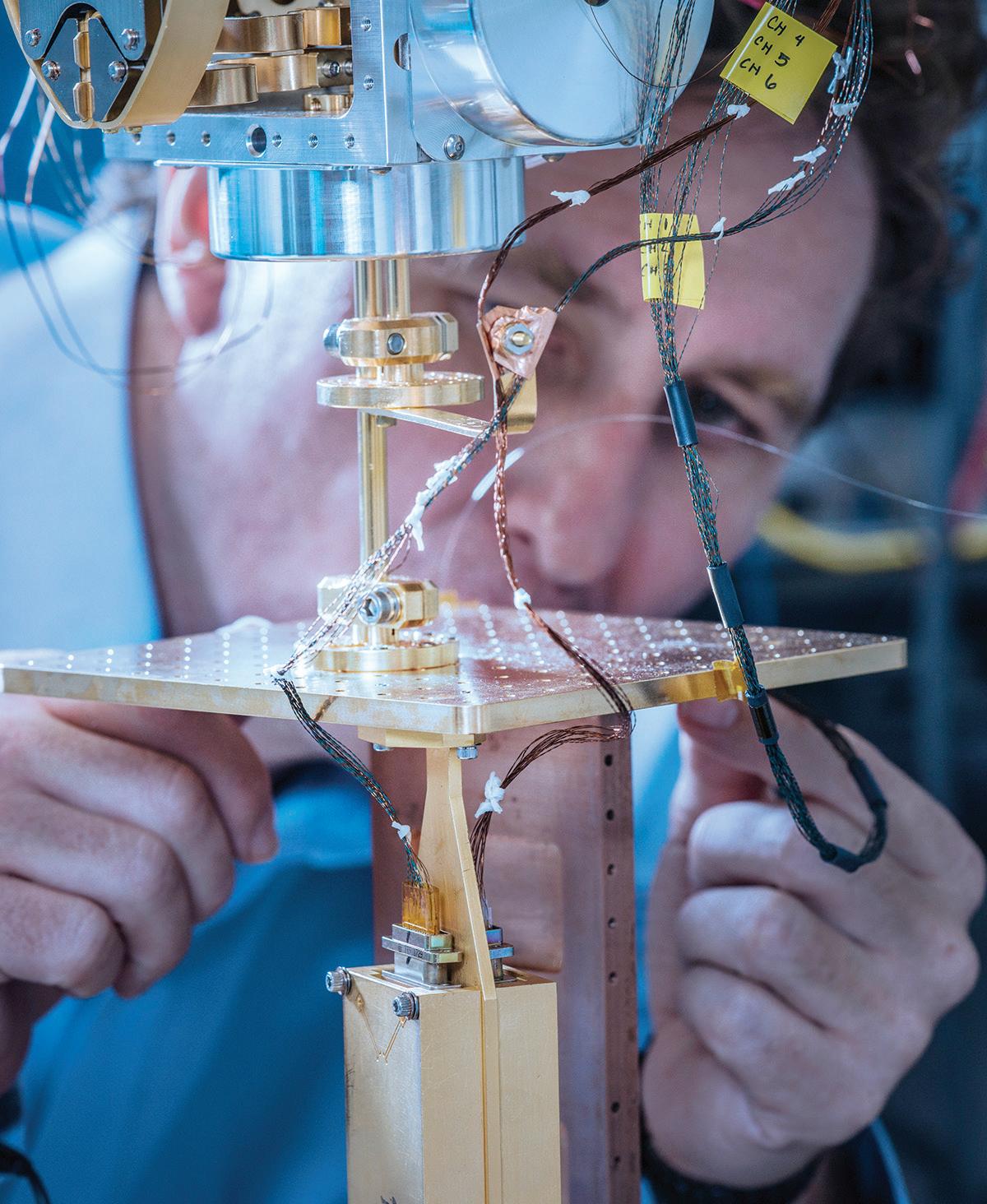
Sadleir, on the other hand, establishes a sensitive quantum state, then exposes it to potential dis turbances from the environment. Measuring how that quantum state breaks down provides valuable information about the source of the disturbance, whether it’s light from an exoplanet, cosmic rays, or other particles, perhaps yet to be discovered.
www.nasa.gov/gsfctechnology
Astrophysicists, including Nobel Laureate Dr. John Mather are always looking to push the limits of light-sensing technology to provide more informa tion about the universe and how it began.
The idea for his sensor derives from the field of quantum computing, where extraordinary ef forts are taken to shield quantum states from any outside interference that could collapse that state.
A Life-Sensing Detector
This illustration shows one concept for a mission to search for signs of life on planets orbiting other stars – the Large UV/Optical/IR Surveyor (LUVOIR) telescope.
erally, we’re not limited to photons,” Sadleir said. “It has applications that require measuring the energy deposited from other particles, such as phonons, electrons, muons, even atoms.”
The search for life elsewhere in the universe is a high priority for NASA – to answer the question, Are we “Ouralone?answer to that question becomes more sig nificant and more impactful the more spectra we can collect,” Sadleir said. “If life signatures can be found, then faster, more efficient collection of light throughout the observation period means increased chances of finding life, as it enables searching a greater number of planets for a greater number of potential life signatures.”
Volume 18 • Issue 4 • Summer 2022 PAGE 5
“A few grams of better detector is equivalent to a few tons of larger telescope, and these detectors will collect spectra all of the time,” Mather said.
Sadleir’s detectors – which measure 20 microns across, or less than a third of the width of a human hair – are special in a few crucial ways.
A Near-Perfect Sensor
Image Credit: NASA’s Goddard Space Flight Center/Conceptual Image Lab
Sadleir’s detector uses materials like gold, which normally never achieve a superconducting state at any temperature. He then uses superconducting leads to take advantage of quantum effects which cause the gold to behave like a weak superconduc tor, sensitive to disruption by single photons.
“The very trait that continues to trouble quantum computing experts today, the fragility of quantum states, we utilize to make sensors with exquisite sensitivity,” Sadleir said. “We are measuring smaller deposited powers and energies than possible before. That is quantum sensing in action: using quantum mechan ics to achieve orders of magnitude improved perfor mance over the existing state of the art.”
Sadleir said these measurements can open a small window to a new understanding of the universe.
He started with a newer technology called a transition-edge sensor which depends on the properties of superconductors at the point where, when chilled, they transition from normal conduc tors to the superconducting state – but with a twist.
technologiesemerginggoddard’sedgecutting•

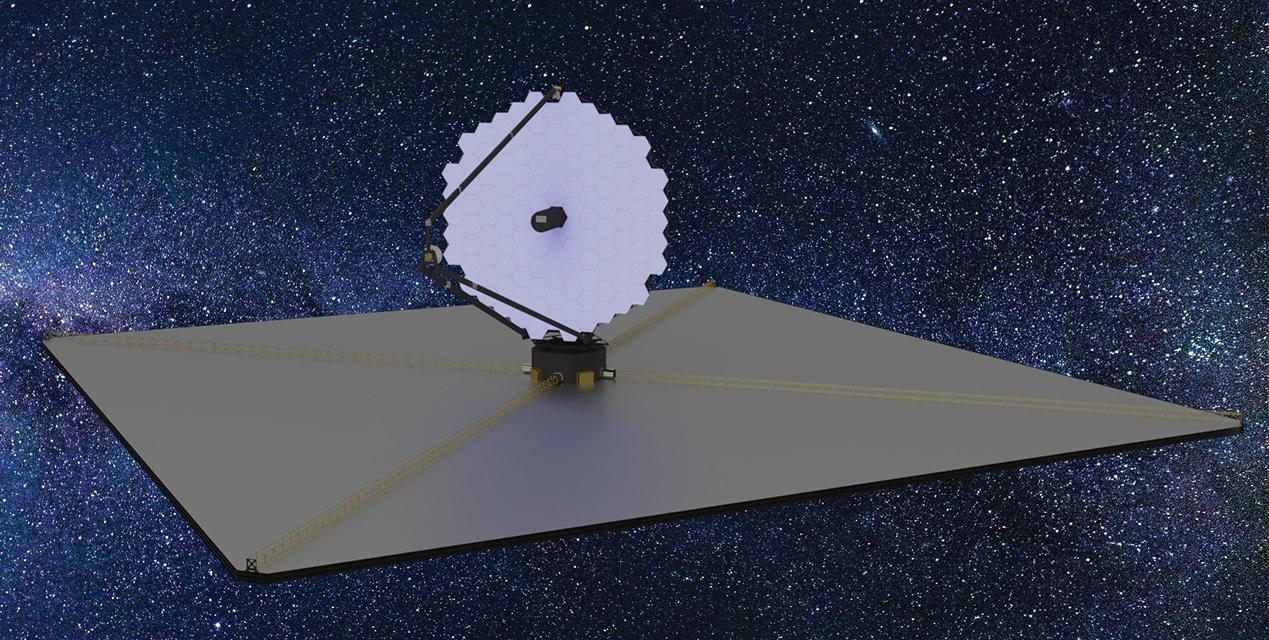
And finally, because each detector is also a spec trometer, Sadleir said they eliminate the need for bulkier and less efficient optical spectrometers.
This artist’s impression shows the planet K2-18b, and its host star. It is the only super-Earth exoplanet known to host both water and temperatures that could support life. Detailed analysis of Hubble image data revealed the molecular signature of water vapor as well as hydrogen and helium in the planet’s
“If planets like Earth are rare, our own world be comes even more precious,” Sadleir said. “If we do discover the signature of life in another planetary system, it will change our place in the universe in a way not seen since the days of Copernicus— placing Earth among a community and continuum of worlds.”
These instruments feed light through a diffraction grating or prism down a long path to separate the wavelengths before the light ultimately reaches a detector array. Which sensor in an array is triggered by a photon after this separation determines its energy, or color, but each step in the process lowers Efficiencyefficiency.iskeyfor a mission that would count individual photons over weeks or even months of “Whenobservations.searching for signs of life,” Sadleir said, “every photon is precious, and we need to efficient ly extract the information contained in each. We need to push the limits, approaching a near-perfect detector system.”
PAGE 6 www.nasa.gov/gsfctechnology
v
CONTACTS John.E.Sadleir@NASA.gov or 301.286.3078 “It’s about collecting spectra,” he added, “and our detectors collect spectra on everything during each phase of the mission.”
atmosphere.ImageCredit:ESA/Hubble,M.Kornmesser
Most light detectors, like those that allow a smart phone camera to take pictures, measure single photons as either an “on” or “off” signal. When a photon hits the sensor and crosses a certain energy threshold, it detects a strike. However, most detectors falsely report photon hits at a rate that can be higher than the number of photons astrono mers expect to capture from very dim exoplanets.
technologiesemerginggoddard’sedgecutting•
Sadleir’s detectors are special in another way. Developed for X-ray astrophysics, they were built to survive the harmful radiation space-based sensors must endure.
Volume 18 • Issue 4 • Summer 2022
For Sadleir – and NASA – answering the big ques tion of life conclusively would be an extraordinary claim, requiring extraordinary evidence.
In addition, these detectors must reset before they can detect another photon strike, potentially missing a “They’redetection.toonoisy to make the measurement,” Sadleir Becausesaid.itmeasures the energy of each photon strike, Sadleir’s detector does not signal false positives. It also performs at an unusually high absorp tion efficiency – the percentage of photons hitting the sensor surface that trigger a detection. His sensors exhibited a 93% or better efficiency for the 1-to 2-micron wavelengths of near-infrared (NIR) light. Other commercially available NIR sensors can have an efficiency as low as 7%. “That misses too many photons at a critical wavelength for the search for life,” he said.
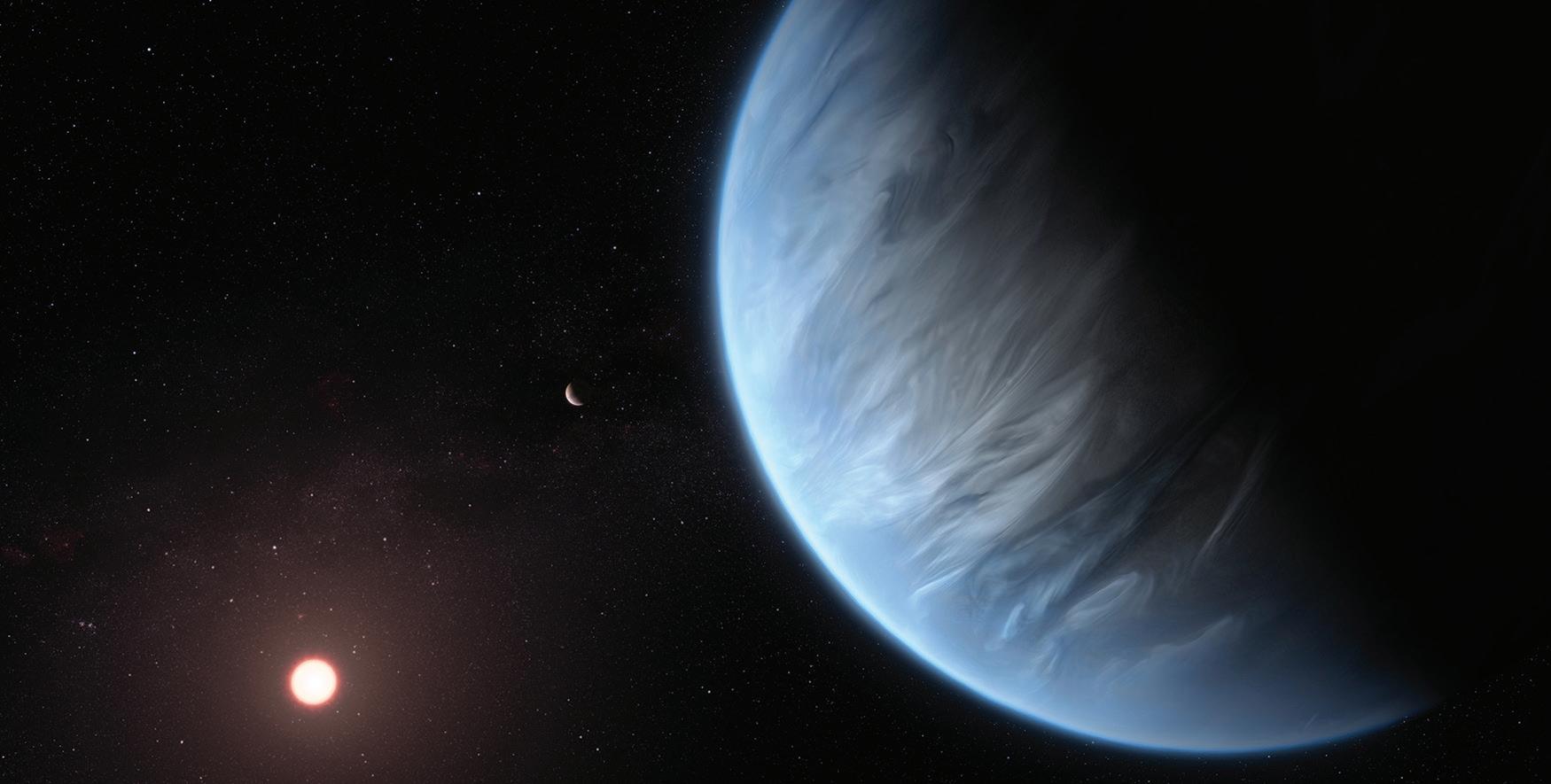
The team’s ideal gravity gradiometer mission would consist of two gravity meters, each consisting of a laser pulsing a cloud of 10 million or more atoms. Flying together in one spacecraft, separated verti cally by up to six feet (two meters), each instrument would measure the subtle differences in gravity –the gradient – between them.
Aquantum technology developed by NASAalong with industry partners could improve the way scientists monitor freshwater resources at the local level by measuring their effects on gravity Earth’s gravity changes slightly from one region to another,and locally, it can change over time Atmosphere, tides, and ocean currents account for much of that change, as well as water moving underground in aquifers, and melting snow and glaciers. Changes in freshwater resources have immediate impacts on the people who depend on them for drinking, farming, or industry. Devel oping methods to monitor and share information about these vital resources is one way NASAhelps improve life on Earth.
NASA Instrument Measures Gravity – By the Atoms
NASACredit:Image
technologiesemerginggoddard’sedgecutting•
www.nasa.gov/gsfctechnology
Interferometry measures a fundamental feature of quantum mechanics where a single particle, electron, photon, ion, or atom can act as a wave, occupying more than one position at any point in time. This quantum state is known as superposition. In their instrument, each atom acts as an interfer ometer, Saif said. The laser pulse reveals detailed information about each atom’s superposition. “With 107 atoms averaged together, this gives you very high-precision measurement,” he said.
Luthcke and Goddard physicist Babak Saif led development of the gravity measuring technology which works by pulsing a laser through a cloud of super-cooled atoms. Mea suring the laser’s interaction with the atoms creates an interference pattern. Scientists know how the atoms should behave at a quantum level, so the interference serves as a real-time measurement of gravity’s pull on the atoms.
Scott Luthcke studies the movement of ground water and glaciers using satellite data and missions designed to measure Earth’s mass.
Volume 18 • Issue 4 • Summer 2022 PAGE 7
TheAtom Interferometer Gravity Gradiometer, developed in partnership with the Fremont, California-based AOSense, offers the potential for accurate, direct, real-time measure ment of Earth’s gravitational field and its changes over time, said Earth scientist Scott Luthcke. (See Cutting Edge, Winter 2019, Page 12).
“One of these instruments in low Earth orbit could advance our current understanding of how Earth’s gravity changes over time and in different regions by an order of magnitude,” Luthcke said. “It’s like being able to improve from looking at large areas, say the size of the entire East Coast, down to the local aquifer level. This is great, not just for science, but as an instrument that provides data for people who do local and regional water management; that’s real societal impact.”


Inc.AOSenseCredit:ImageCredit:Image
Traditionally, missions compile those maps by close orbiting closely over time and inferring grav itational forces from spacecraft positioning data.
In addition to mapping worlds like these more quickly or even whole solar systems, Saif said, the AIGG instrument could someday be used to read any gravity signature – including the 96 percent of our universe that is unknown dark matter or dark energy
technologiesemerginggoddard’sedgecutting•
www.nasa.gov/gsfctechnology
Volume 18 • Issue 4 • Summer 2022
Navigating Other Worlds AIGG has applications across planetary explora tion as well, Saif said. The Gravity Recovery and Interior Laboratory (GRAIL) mission mapped the Moon’s gravity, providing insight into our natural sat ellite’s structure.AnAIGG probe of the Moon or Mars could locate underground networks of lava tubes – a potential target for explora tion and source of shelter.
Precise gravitational maps of other moons, asteroids or other small bodies could improve safety and precision of future land ing or sample missions.
The Origins, Interpretation,SpectralResource Identification, SecurityRegolith Explorer (OSIRISREx) spacecraft orbited the near-Earth asteroid Bennu almost two years before selecting a sample site: precision mapping the rocky asteroid and its lowgravity environment. Saif said their instrument could provide an accurate map within months or less, depending on the object.
“The best part is, there is no telescope is required,” he said. “Our system is completely closed – you’re PAGE 8 reacting to the gravity field directly, and the mea surement is all internal. You don’t have to measure distance to another spacecraft, or object, or read the position of stars. From the point of view of radia tion, it’s better too, because your instrument can be shielded from interference; none of it is exposed to the environment.”
The Atom Interferometer Gravity Gradiometer, shown here at the AOSense Lab in Fremont, California, directly measures changes in gravity’s effect on atoms suspended in a cloud at super-cold temperatures.
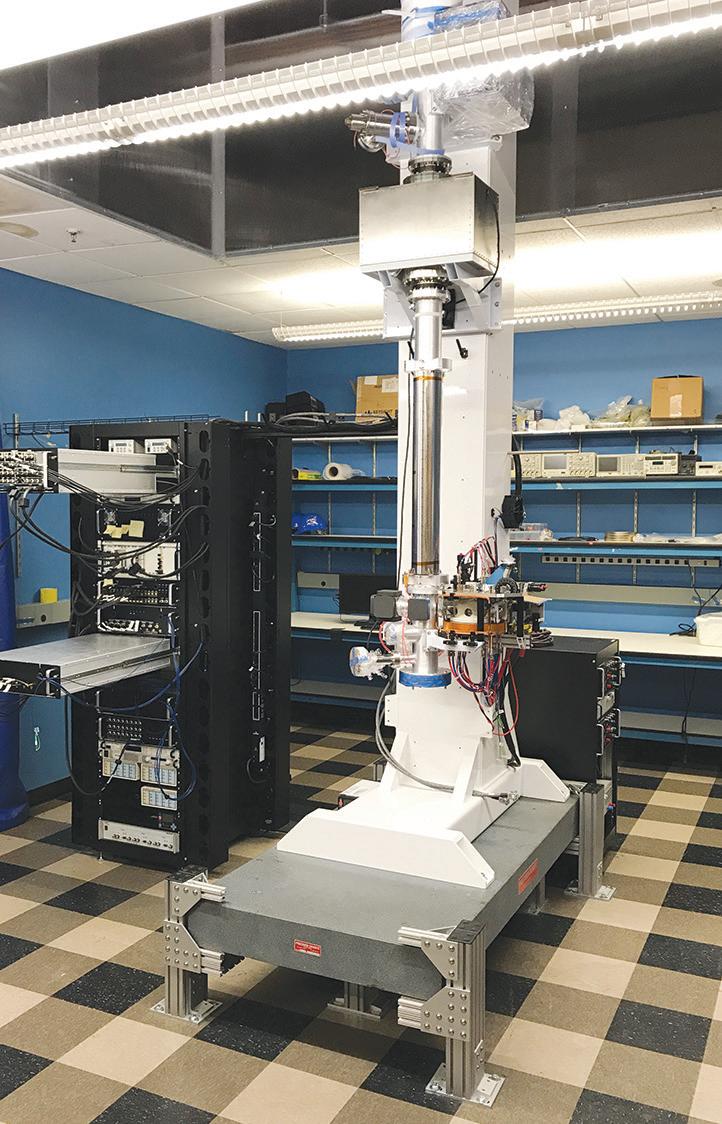
“Now you’re talking about mapping the change in gravity even every few days,” Luthcke said, “and at a high spatial resolution. You’re not just calculating how deep the water is by measuring individual wells; you’re really measuring the change in the mass, where the water moves in the earth. Imagine understanding the mass change at the size of individual glaciers and ice-drains. Imagine being able to predict what will happen with those currents or aquifers or glaciers in the next few days or weeks like we do now with weather.”
Volume 18 • Issue 4 • Summer 2022 PAGE 9
Radar and lidar altimetry missions measure the round-trip travel time of photons bouncing back from Earth’s surface, revealing elevation, tree canopy, ice sheet height, and changes of mass over time. More recently, NASA’s Gravity Recovery and Climate Experiment (GRACE) and the GRACE
CONTACTS Bringing it Home
Satellite Geodesy – Innovation Born at Goddard
www.nasa.gov/gsfctechnology
Follow-On mission used range measurements between two precision-orbiting spacecraft to show how gravity changes from month to month. These missions showed changes in mass to Earth’s ice sheets over time, as well as changes in groundwa ter distribution over their 20-year record.
With all the applications for exploration, Luthcke said he doesn’t want to get distracted from the “singular thing that would have the most impact for humans – which is to put one of these instruments in low Earth orbit.”
“We’ve got something right in front of us that can vastly improve our understanding and model of the time variable gravity field of Earth,” he said. “That would benefit people everywhere that depend on effectively managing Earth’s precious water resources.” v
“I just think it’s really cool, that satellite geodesy of Earth really started here at Goddard,” Earth Scientist Scott Luthcke said. “If you look at your phone and it tells you where you are, that’s the result of satellite geodesy.”
v
The Gravity Recovery and Climate Experiment - Follow On mission continues the legacy of GRACE, tracking Earth’s water movement and surface mass changes across the planet. Image Credits: NASA/JPL-Caltech
Scott.B.Luthcke@NASA.gov or 301.614.6112 Babak.N.Saif@NASA.gov or 301.286.5433
Earth scientists have measured our planet’s mass and shape from orbit since the first satel lites returned precise data about gravity's effects on their position and velocity. In 1955, Dr. John A. O’Keefe formally proposed using this data to infer Earth’s shape by calculating gravity’s influence on the spacecraft.America’s second successful satellite, Vanguard 1, provided the tracking data scientists used to calculate that Earth is ever so-slightly pear-shaped. Since then, countless missions’ data added to this model of Earth in a field called satellite geodesy: a fundamental necessity for working global positioning systems
To improve resolution of these water resources down to smaller scales and shorter time intervals, theAtom Interferometer Gravity Gradiometer (AIGG) directly measures gravity’s pull against free-falling atoms, Goddard physicist Babak Saif “said.Usually, when we do gravity measurements on the science side,” he said, “we don't measure the gravity. We always measure something else and then infer gravity. WithAIGG, you’re actually measuring the behavior of the atoms as they are falling, when they’re not being affected by anything but gravity.”
To prove their instrument, Saif and Luthcke said they would like to combine data from a scaled-down gradiometer with a GRACE-successor mass measurement mission like NASA’s Mass Change future mission, an integral part of the Earth System Observatory. Adding a gradiometer to the mass change mission would enhance the science return for a relatively small additional cost.
technologiesemerginggoddard’sedgecutting•

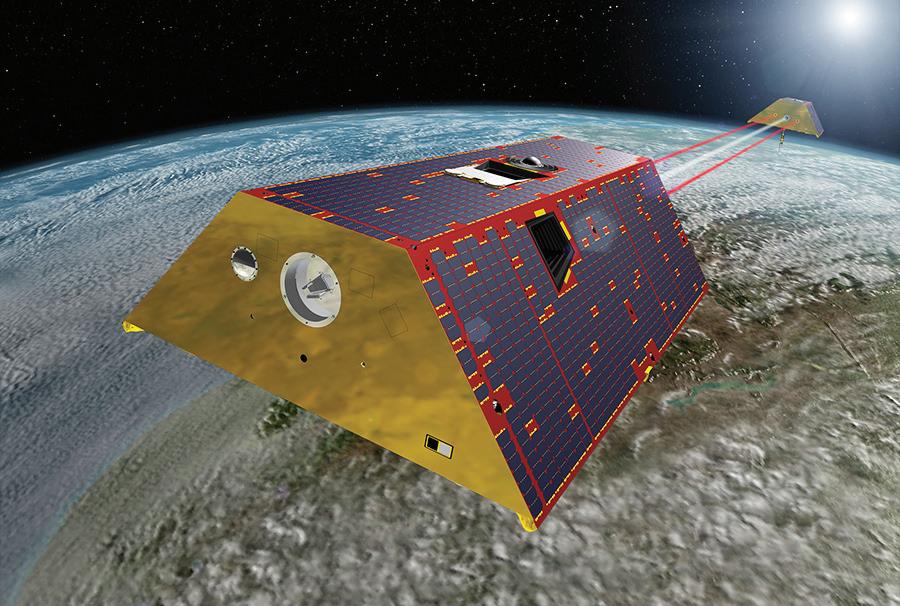
Transmitting an entire message through entangled photons is a long way off Goddard physicist Babak Saif said. “Right now, optical communications infrastructure does not even exist,” he said. “These technologies are still in the testing and demonstra tion phases. Optical communications performance metrics come nowhere close to any of the specifi cations needed for quantum communications.”
Uniquely Situated Goddard’s Exploration and Space Communications (ESC) projects division, part of NASA’s Space Com munications and Navigation (SCaN) program office, leads the way in developing a high-speed laser communications capability that could enable further advances in quantum communications.
SCaN’s proven track record of high data-rates and near-zero downtime is driven by NASA’s responsi bility for keeping astronauts, the International Space
Quantum Communications – Developing Capabilities for the Future
Creating a reliable, high-speed quantum communica tions network faces two critical technology gaps, said PAGE 10 Harry Shaw, Goddard staff engineer for the Telecom munications and Network Technologies Branch. No one has yet developed a reliable quantum memory, and quantum-encoded data cannot be cloned or copied without collapsing the quantum state.
Volume 18 • Issue 4 • Summer 2022
NASACredit:Image
To create entangled pairs, lasers pump high energy photons through a specialized crystal that can emit two lower-energy photons for about one in a trillion photons received. Experiments to date have transmitted a few kilobits per second of entangled photon pairs, enough to create an encryption key to be distributed to a sender and receiver That infor mation is used to encrypt and decrypt information sent in classical bits: ones and zeroes.
Instant, secure commu nications anywhere – the promise of
technologiesemerginggoddard’sedgecutting•
“There are some technology bridges that have to be built and crossed to enable practical quantum communications,” Shaw said. “Until those are solved, it’s going to be very interesting, but the ap plications will be limited.”
someone eavesdropping on a communication, collapses the quantum state of both instantaneously into a single, or distinct, state.
states,onebyevenpartwhateverasinWhensimultaneously.moreperpositionexistthatertytionsQuantumcriticalracingnessesgovernmentscommunicationsquantumhasandbusiaroundtheworldtoperfectthistechnology.communicadependsonapropofquantumphysicsallowsaphotontoinastateofsu–occupyingthanonelocationaphotonexiststwolocations,knownanentangledpair,affectsonealsoaffectsitsmatewhenseparateddistance.Measuringofapair’squantumforinstanceby
www.nasa.gov/gsfctechnology
The Low-Cost Optical Terminal enables communications from laser terminals in orbit or as far as the Moon.
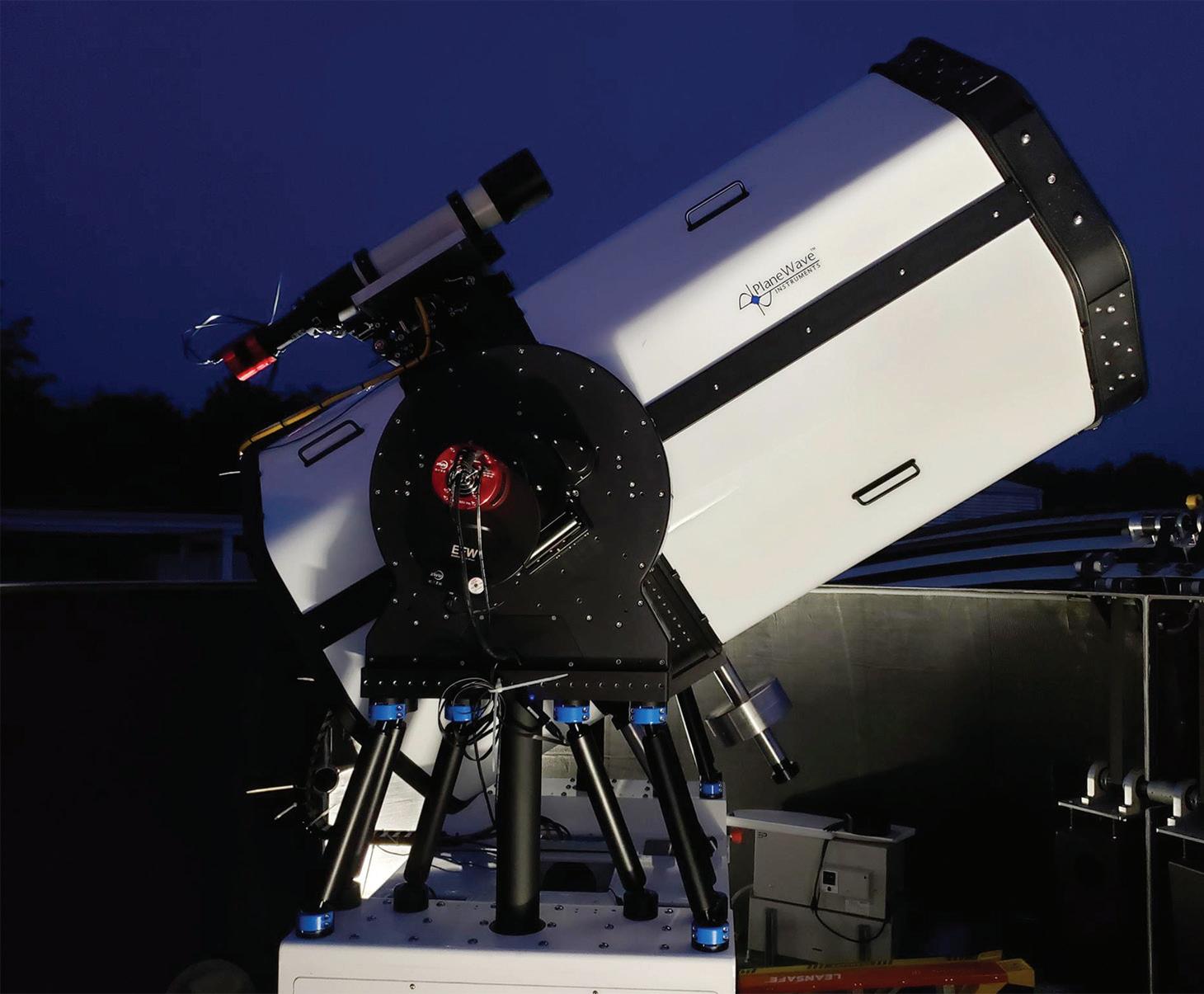
RyanNASA/DaveCredit:Image
and ESC launched the TeraByte InfraRed Delivery (TBIRD) payload – a small satel lite demonstrating NASA’s highest-ever optical data rates at 200 gigabits per second, and the Laser Communications Relay Demonstration (LCRD) –the agency’s first-ever optical relay. They are also developing a laser communications terminal for the International Space Station and the crewed Artemis II Moon mission. These innovations synergize with developments in quantum communications Saif said. Both classical and quantum communications depend on information transmitted by photons. This means research and development into quantum communications stands to drive improvements to laser communications using classical data transmission as well, he said.
Several new initiatives could bring the state of the art closer to the needs of future quantum networks.
technologiesemerginggoddard’sedgecutting•
To enhance reception for optical receivers like LCOT, Goddard physicist Aaron Yevick success fully tested an advanced form of adaptive optics, or image correction technology needed to overcome distortion created by Earth’s turbulent atmosphere. Yevick used an optical fiber device called a photonic lantern, to receive and synchronize multiple signals
The Laser Communications Relay Demonstration (LCRD) communicates data on a beam of light between the International Space Station and ground stations like this one in Hawaii.
“LCOT promises to be a powerful new facility for use in laser communication and other research right here in our backyard,” Lafon said. “Optical and quantum communications will be primarily what we do, but we have gotten a lot of interest in using it for lidar work, debris tracking and mitigation efforts, and other things. Since we designed it to be modular and highly reconfigurable, it can easily be set up to accomplish multiple objectives.”
www.nasa.gov/gsfctechnology Station, and fleets of satellites orbiting Earth safe and Recently,connected.SCaN
Volume 18 • Issue 4 • Summer 2022 PAGE 11
Goddard Optical Physicist Robert Lafon recently helped complete a Low-Cost Optical Terminal, or LCOT, for laser communications downlinks. Similar to Optical Ground Station 2 at Haleakala, Hawaii, and JPL’s Optical Communications Telescope Laboratory (OCTL) on Table Mountain, California, LCOT’s 70 cm collecting mirror at the Goddard Geophysical and Astronomical Observatory (GGAO), enables communications at least as far as the Moon and is capable of tracking relatively faster moving satellites in low Earth orbit. Another terminal is under development at White Sands in Las Cru ces, New Mexico.

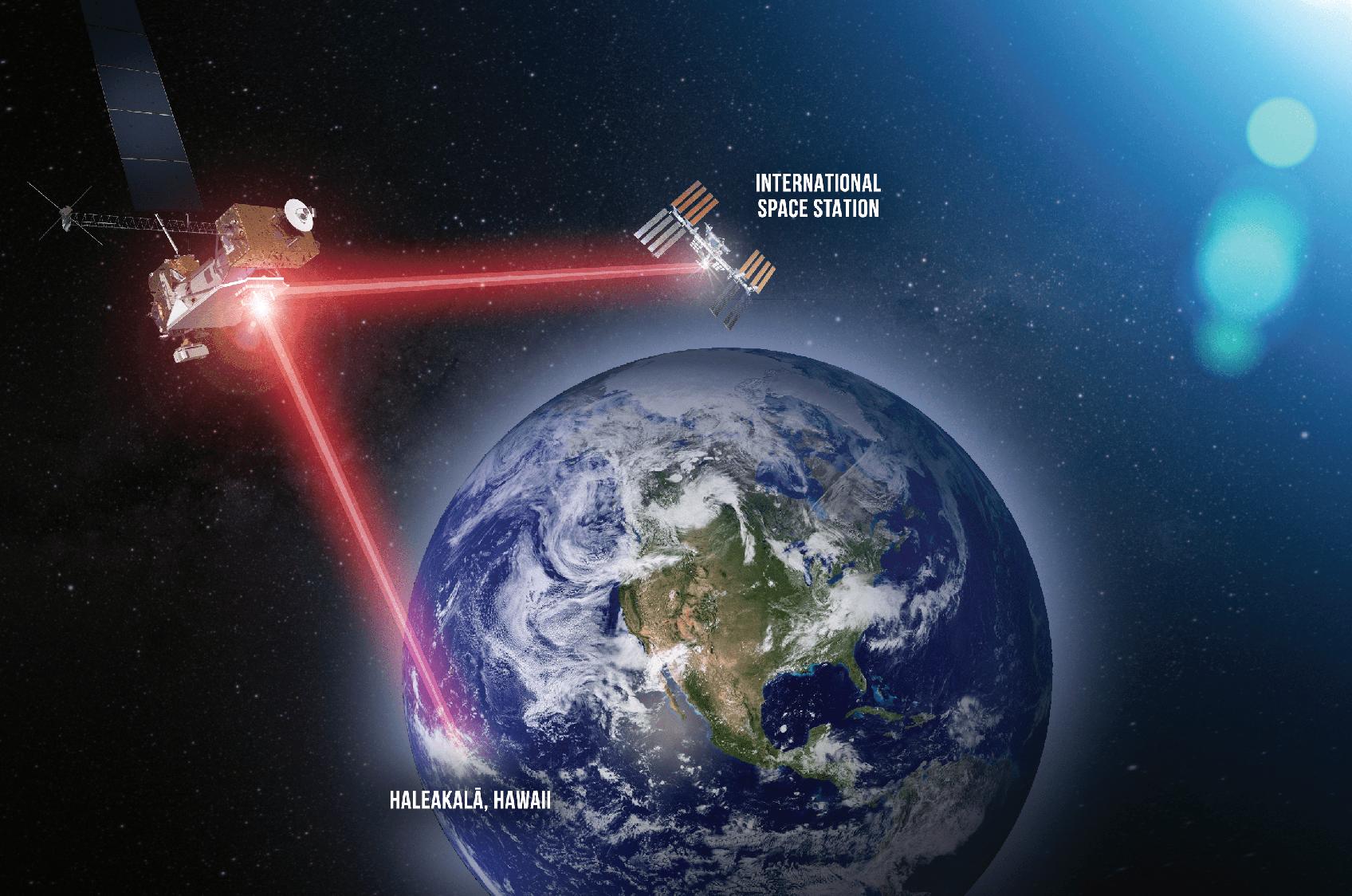
Image Credits: NASA Goddard/Sandra Vilevac
ACCESS’s quantum lab is expanding to include experiments with orbital angular momentum (OAM) entanglement. “Orbital angular momentum allows encoding information in very high-density codes,” Shaw said, “and they will be using this OAM property to experiment with encrypted communications.”
Over the past six years, theproject team has built a community of Ph.D.’s with ex pertise in the field while expanding Goddard’s laboratory capacity for developing quantum communications solutions.
Harry.C.Shaw@NASA.gov or 301.286.8680 from a single distorted source. The technology accurately records the wave phase of each signal. Then his team digitally processes these signals into a coherent, single phase.
CONTACT
Volume 18 • Issue 4 • Summer 2022
efficiency is what everybody’s working towards.”
“We have to grow the pool of researchers with the skills to be able to develop the quantum memories and repeaters and relays and entanglement sources and all the other things we need,” he said. v
Communicating and navigating accurately through space also requires extremely precise, synchro nized clocks. Space-based clocks like those used in global positioning networks must be accurate down to 3 nanoseconds and require complex protocols to synchronize over vast distances. Multi-party quantum clock simulation uses entangle ment to eliminate the time lag caused by distance and simplify those protocols. Shaw is working with a team from Tulane University in New Orleans to develop a lab test for a quantum synchronization protocol they created. v
Growing Talent for Quantum Investigations
“The ACCESS quantum lab enables graduate students to do quantum experiments as part of their dissertation research,” Shaw said, “while supporting breakthroughs for our academic partners’ research.
Shaw’s work with graduate students interested in quantum physics is about more than just keeping abreast of the emerging technology. It’s about developing a community of expertise with an affinity for Goddard. That doesn’t mean they’ll come work for NASA, but the affiliation can’t hurt, he said.
Shaw’s students use commercially available quantum photon sources in two wavelengths of infrared and near-infrared light to experiment with two types of superposition or entanglement: polarization, the orientation of light’s electric field, and energy/time entanglement.
Harry Shaw, Goddard staff engineer for the Telecommunications and Network Technolo gies Branch, works with graduate students from around the country to conduct quantum communications research aimed at bridging gaps in existing technology. He is a member of theAdvanced Communications Capabilities for Exploration and Science Systems (ACCESS) project.
“These technologies provide a testbed for Goddard to do classical, and eventually quantum optical com munications,” Shaw said. Shaw also hopes to test quantum photon detectors designed by Goddard physicist Jack Sadleir to eval uate their suitability for a quantum-communications receiver.
“It’s possible that this detector, designed to look for signs of life on planets outside our solar system, could find applications in detecting and characterizing quantum-entangled photons,” Shaw said. (See story, page 4) “High-speed, single-photon detectors with low false positives and high quantum
technologiesemerginggoddard’sedgecutting•
Harry Shaw, top center, mentors interns working on theoretical quantum communica tions experiments.
PAGE 12 www.nasa.gov/gsfctechnology
“We have some interesting theoretical work that we’re trying to take from theory and work out on the bench,” Shaw said.
It also broadens diversity in STEM participation by bringing on more women and minorities into the quantum arena through internships and new hires.”

Sultana gets her dots, which vary from 2 to 10 nanometers or less than 50 atoms thick, from the lab of chemistry professor Moungi Bawendi, at the Cambridge-based Massachusetts Institute of Technology She then uses them to break down light from a planet or other target into portions of the spectrum, creating a sort of fingerprint which reveals what elements or compounds that light has touched.
Mahmooda Sultana investigates new applications of quantum physics using nano-scale materials with unique characteristics that make them particularly attractive for spaceflight uses.
www.nasa.gov/gsfctechnology
Goddard instrument sci entist Mahmooda Sultana developed the Quantum Dot Spectrometer to help Quantum dots are a type of semi conducting nanocrystal that absorbs and re-emits different wavelengths of light depending on their size, shape and chemical composition (See CuttingEdge, Winter, 2017, Page 11).
technologiesemerginggoddard’sedgecutting•
Atypical spectrometer can be a relatively bulky piece of equipment, taking up precious real estate aboard a satellite. What sets Sultana’s Quantum Dot Spectrometer apart is a literally small miracle of modern chemistry.
In NASA’s hunt for water and resourc es beyond Earth, a new technology could coat the “skin” of a satellite turn ing its entire surface into a sensor that tallies the chemicals present on distant planets. Solving the mysteries of our home planet, solar system, and beyond is a key priority for NASA, and the new sensor could be a powerful tool in the investigation.
Vials of quantum dots glow in different colors under ultraviolet light. The colors of light emitted are determined by the nano-scale size and compo sition of the crystals in suspension.
As an engineer interested in planetary science, Sultana said her quantum dot spectrometer could identify water and other chemicals in lunar soil and characterize surface and atmospheric elements of other planets
“The math is complex,” she added, “but using machine learning, we are able to get amazing ac curacy, even on more complex spectral curves.”
Quantum Dots Enable Spacecraft-as-Sensor Concept
Volume 18 • Issue 4 • Summer 2022 PAGE 13
“Basically, we are converting the entire optical problem into a math problem,” Sultana said. “The dots can be identified in the lab to register light of a particular wavelength – a fraction of the chemi cal fingerprint. Detectors on the other side of the dots collect the fractions, then the data is handed over to computers on the ground to reassemble the complete fingerprint.”
Image courtesy: Mahmooda Sultana
IzzoNASA/PatCredit:Image
Enabling new applications on SmallSats as well as solar sails, Sultana’s instrument has potential for studying Earth’s surface composition, ocean color, vegetation, and atmospheric chemistry, as well as providing insight into auroral interactions.

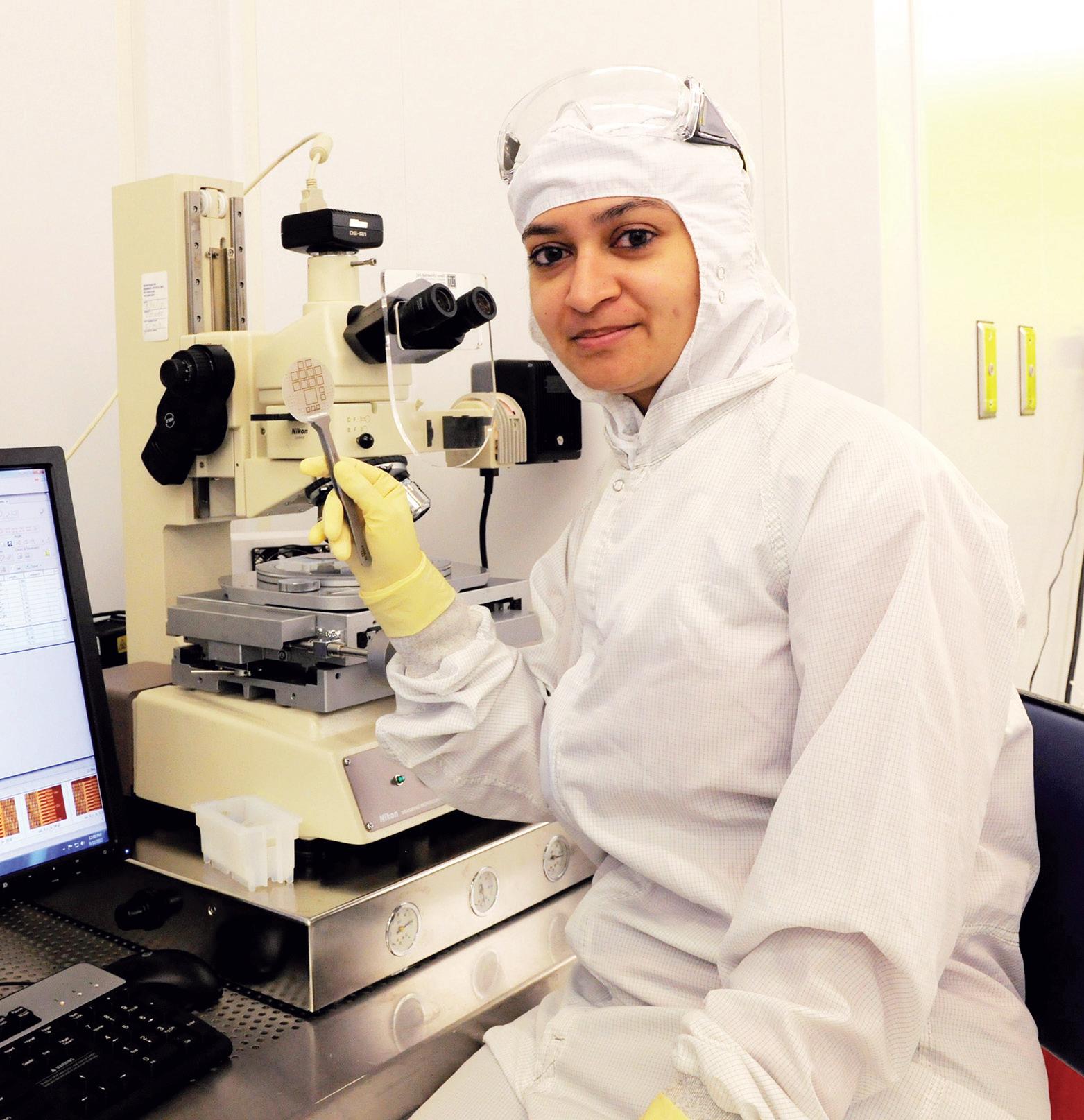

The sail – driven by the gentle pressure of sunlight
CONTACT Mahmooda.Sultana@NASA.gov or 301.286.6973 across a large area, the sail would pick up speed by orbiting close to the Sun, propelling it into the outer solar Oncesystem.there,Sultana’s vision could accomplish critical science goals, Goddard planetary scientist Conor Nixon said.
Image Credits: NASA, ESA, CSA, and STScI
“There is currently a gap for exploring the NeptuneTriton system,” he said. “Can we do some Neptune science on a fast-flyby, lower-cost mission? It’s the sort of planetary system that SCOPE could find a niche in exploring.”
“This is a game-changing concept,” she said. “We are essentially addressing three of the key barriers to outer solar system exploration: the high cost, long travel time, and a narrow window to launch a mis sion that can rendezvous with these distant planets.”
technologiesemerginggoddard’sedgecutting•
The versatility of the quantum dot technology could enable a low-cost mission to an outer solar system
“As people around the world develop the capability to print electronics or produce different materials and structures, more instruments like the Quantum Dot Spectrometer can be printed directly with the solar sail to create additional ScienceCraft opportunities,” Sultana said. v
Splanet.ultana’s concept – ScienceCraft for Outer Planet Exploration, or SCOPE – capitalizes on the sensor’s versatility and low mass.Asolar sail, printed with separate layers for readout electronics, detector ar ray, quantum dot spectrometer, and microlens array, would serve as a spacecraft, propulsion system, and science instrument in one.
PAGE 14 www.nasa.gov/gsfctechnology
Data from spectrometers is displayed as a spectrum, as in this image capturing the signature of water vapor, clouds and haze from exoplanet WASP-96 b. Coming from a puffy gas giant orbiting a star 1,150 light years away, the blue line shows peaks where specific colors (wavelengths) of light are bright and valleys where colors are dim or absent. Each element or chemical compound contributes its unique spectral curve that serves as its “signature” within the bigger picture.
In 1989, Voyager 2’s close-up images of Triton first revealed its icy surface, marked by plumes that hinted at an active geology and a hidden ocean be neath the ice. Investigating how Triton has changed over time would help scientists better understand how solar system bodies evolve and function.
Sultana calls this vision for future exploration vessels ScienceCraft.
ScienceCraft – a New Vision for Exploration
Sultana is working to develop this mission concept through a Phase I NASAInnovativeAdvanced Concepts (NIAC) Program award granted thisApril. Her team has already automated the dot printing process, and the sail is being developed by coinvestigatorArtur Davoyan, a professor at UCLA.
Volume 18 • Issue 4 • Summer 2022

Traditional lasers generate light by exciting an elec tron within an atom’s outer shell, which then emits a single photon as it transitions – or returns to its resting energy level. Different atoms produce differ ent frequencies of light based on the fixed amount of energy it takes to excite one electron. However, lasers fall short in a particular portion of the spec trum between infrared and microwave known as the terahertz gap.
Laser to Find Water on the Moon
Berhanu Bulcha shows off his terahertz laser technology in his lab at NASA’s Goddard Space Flight Center in Greenbelt, Md.
Finding water on the Moon could be easier with a Goddard laser that capitalizes on an effect called quantum tunneling to generate a high-powered beam in a bandwidth where water is known to absorb light. Locating water and other resources is a NASA priority crucial to exploring Earth’s natural satellite and other objects in the solar system and beyond. Previous experiments inferred, then confirmed the existence of small amounts of water across the Moon. However, most technologies do not distinguish among water, free hydrogen ions, and hydroxyl, as the broadband detectors used cannot distinguish between the different volatiles.
Electromagnetic oscillators like those that generate radio or microwave frequencies produce low-pow ered terahertz pulses by using a series of amplifiers and frequency multipliers to extend the signal into
Tiny, High-Powered
“The problem with existing laser technology,” Dr. Bulcha said, “is that no materials have the right properties to produce a terahertz wave.”
GiuntoMichaelCredit:Image
As the name implies, spectrometers detect spectra –wavelengths of light – in order to reveal the chem ical properties of matter that light has touched. Most spectrometers tend to operate across broad sec tions of the spectrum. Heterodyne instruments dial in to very specific light frequencies such as infrared or terahertz. Hydrogen-containing compounds like water emit photons in the terahertz frequency range –2 trillion to 10 trillion cycles per second – between microwave and infrared. Like a microscope for subtle differences within a bandwidth like terahertz, heterodyne spectrome ters combine a local laser source with incoming Mlight.easuring the difference between the laser source and the combined wavelength provides accurate readings between sub-bandwidths of the spectrum.
Goddard engineer Dr. Berhanu Bulcha said a type of instrument called a heterodyne spectrometer could zoom in on particular frequencies to definitively identify and locate water sources on the Moon. It would need a stable, high-powered, terahertz laser which was prototyped in collaboration with Longwave Photonics through NASA’s Small Business In novation Research (SBIR) program.
“This laser allows us to open a new window to study this frequency spectrum,” he said. “Other missions found hydration on the Moon, but that could indicate hydroxyl or water. If it’s water, where did it come from? Is it indigenous to the formation of the Moon, or did it arrive later by comet impacts? How much water is there? We need to answer these questions because water is critical for survival and can be used to make fuel for further exploration.”
Volume 18 • Issue 4 • Summer 2022 PAGE 15
technologiesemerginggoddard’sedgecutting•
www.nasa.gov/gsfctechnology


CONTACT Berhanu.T.Bulcha@NASA.gov or 301.286.7418
GiuntoMichaelCredit:Image
technologiesemerginggoddard’sedgecutting•
CuttingEdge is published quarterly by the Office of the Chief Technologist at NASA’s Goddard Space Flight Center in Greenbelt, Maryland. The publication describes the emerging, potentially transformative technologies that Goddard is pur suing to help NASA achieve its missions. For more information about Goddard technology, visit the website listed below or contact Chief Technologist Peter Hughes, Peter.M.Hughes@NASA.gov. If you wish to be placed on the publication’s distribution list, contact Editor Karl Hille, Karl.B.Hille@NASA.gov. Publication Number: NP-2022-8-833-GSFC the terahertz range. However, this process con sumes a lot of voltage, and the materials used to amplify and multiply the pulse have limited efficien cy. This means they lose power as they approach the terahertz frequencies. From the other side of the terahertz gap, optical lasers pump energy into a gas to generate photons. However, high-powered, terahertz-band lasers are large, power hungry, and not suitable for space exploration purposes where mass and power are limited, in particular hand-held or small satellite applications. The power of the pulse also drops as optical lasers push towards the terahertz bandwidths. To fill that gap, Dr. Bulcha’s team is developing quantum cascade lasers that produce multiple pho tons from each electron transition event by taking advantage of some unique, quantum-scale physics of materials layered just a few atoms thick. In these materials, a laser emits photons in a specific frequency determined by the thickness of alternating layers of semiconductors rather than the elements in the material. In quantum physics, the thin layers increase the chance that a photon can then tunnel through to the next layer instead of bouncing off the barrier. Once there, it excites additional photons. Using a generator material with 80 to 100 layers, totaling less than 10 to 15 microns thick, the team’s source creates a cascade of terahertz-energy photons. This cascade consumes less voltage to generate a stable, high-powered light. One drawback of this technology is its beam spreads out in a large angle, dissipating quickly over short distances. Using innovative technology supported by Goddard’s In ternal Research and Development (IRAD) funding, Dr. Bulcha and his team integrated the laser on a waveguide with a thin optical antenna to tighten the beam. The integrated laser and waveguide unit re duces this dissipation by 50% in a package smaller than a quarter He applied for Research Opportunities in Space and Earth Sciences (ROSES) funding to con tinue the work and prepare a flight-ready laser for NASA’sArtemis program. The laser’s low size and power consumption allow it to fit in a 1U CubeSat – about the size of a teapot along with the spectrometer hardware, processor, and power supply. It could also power a handheld device for use by future explorers on the Moon, Mars, and beyond.
Volume 18 • Issue 4 • Summer 2022
PAGE 16 www.nasa.gov/gsfctechnology
v
This tiny laser capitalizes on quantum-scale effects of materials just tens of atoms across to generate a high-powered beam in a portion of the spectrum where traditional lasers fade in strength.
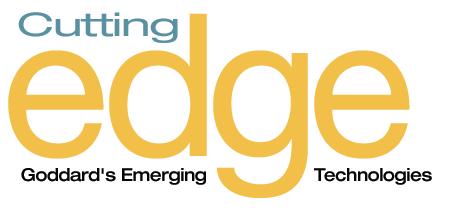
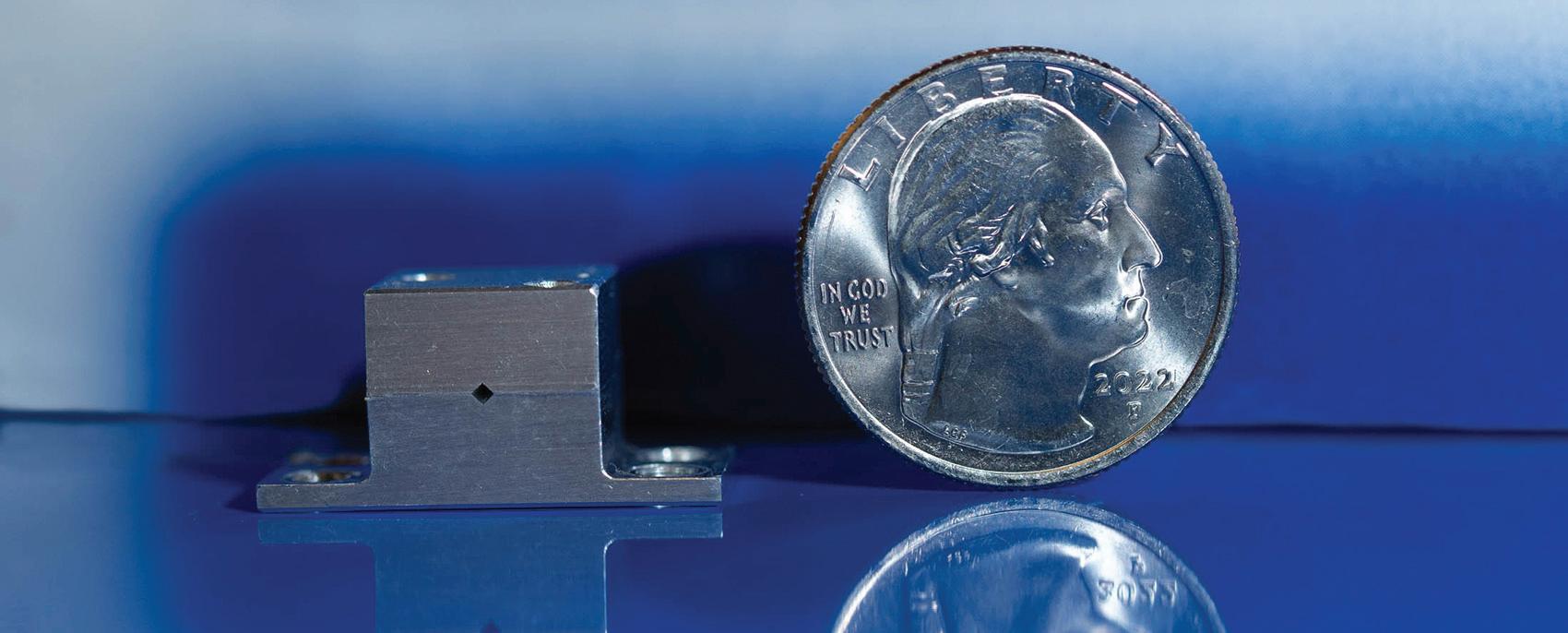